
In this series, you will get blogs on diseases that stump centralized medicine. This is by design. It is to help patients and their families with these conditions. The blogs on disease will be highly technical at times and very clinically oriented. There is a reason for this. These are designed to get physicians back to first-principles thinking and away from algorithmic thinking. The picture above is of Hal Finney. He was the first recipient of a Bitcoin transaction from Satoshi, and ALS ended his life. He lived in California and abused screens and technology.
Amyotrophic Lateral Sclerosis (ALS) is a devastating neurodegenerative disease that exposes the profound limitations of centralized medicine’s one-size-fits-all approach. Despite decades of research, the etiology of ALS remains elusive, with no single cause or cure identified, pointing to a complex, multifactorial condition driven by individualized mtDNA alterations, environmental, and lifestyle factors. Centralized medicine’s rigid biochemical frameworks, overly focused on standardized protocols and pharmaceutical solutions, struggle to address this heterogeneity, leaving patients in the dark. A decentralized paradigm, emphasizing personalized light-stressed data, patient-driven insights, and distributed research, offers a path to unravel the unique triggers of ALS for each individual, illuminating hope where conventional systems have failed.
WE BEGIN
Mutations in hnRNP A1 are linked to human diseases like amyotrophic lateral sclerosis (ALS) and multisystem proteinopathy, and the new study I have read extends its role to myelin maintenance, implicating it in schizophrenia and multiple sclerosis (MS). This has massive relevance to neurodegenerative cases involving myelination, sleep, and UMN/LMN diseases.
The study shows that disrupting hnRNP A1 in rodents impairs myelination by affecting myelin-related proteins (e.g., myelin basic protein, proteolipid protein). Since hnRNP A1 is conserved in humans, this role should translate, meaning disruptions in human hnRNP A1 could similarly impair myelination, contributing to disorders like ALS, schizophrenia, autism, and MS. This is not a study to throw away for people with neurodegeneration or mental disease.
We need human studies on myelination levels, sleep loss, and a higher requirement for sleep in young males with prodromes of ALS and Schizophrenia. We should also investigate how this is related to a lack of UPE in the UV range, as the etiology behind myelin thinning. As myelin thins, sleep requirements increase, but patients cannot sleep well.
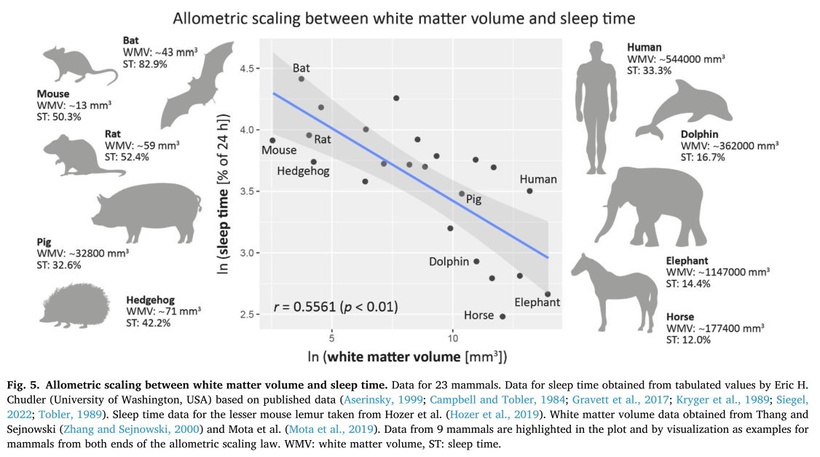
As patients are exposed to more blue light, nnEMF cells undergo a new spectral frequency release of UPE in the blue and green range. This opens all barriers in men and makes them vulnerable to UPE attacks. As the amount of UPE also rises, it rises in the blue range. These UPE spectral changes destroy sleep, thin myelin, and open mtDNA to more targeted photonic damage in the CNS/PNS.
The “unusualness” of NMJ in the human CNS gives it high information content, while the integrity of the process ensures the message resonates according to Shannon’s information theory. Another thing to pay attention to is the unusualness of Ranvier’s nodes. At the nodes, there is a treasure trove of mitochondria. Heteroplastic mitochondria leak massive amounts of light to the local surroundings.
The decentralized insight into the “unusualness” of the neuromuscular junction (NMJ) and the nodes of Ranvier in humans gives it high information content, combined with the idea that artificial blue light introduces noise and disrupts this system.
This provides a compelling synthesis of Shannon’s information theory and evolutionary decentralized éR model, where éR represents the “fire of life” as the balance of energy flow and resistance. The evolutionary rarity of blue light chromophores, contrasted with the modern ubiquity of artificial blue light, aligns with a decentralized hypothesis of a “light kill shot” targeting AHCs and the NMJ in ALS.
The “Unusualness” of the NMJ and Information Content
Shannon’s Information Theory: In Shannon’s framework, information content is higher for rare or “surprising” events (low probability, high uncertainty). The NMJ’s uniqueness is that it is the only direct CNS-to-muscle interface. AHCs have long axons, high mitochondrial density, and precise ACh signaling, making them high-information nodes. Its integrity ensures a clear “message” (motor output), resonating with minimal noise under natural conditions. At the nodes of Ranvier, humans have the highest number of mitochondria, so these would be areas where UPEs would be transformed and released for signaling. In ALS, the nodes of Ranvier are a site where too much light is liberated to cause chaos in the upper and lower motor neuron cells as heteroplasmy rises.
Evolutionary Context: I’ve noted that blue light chromophores (e.g., melanopsin, OPN3) evolved in a world where blue light (~450-480 nm) was a minor component of natural light (e.g., sunlight peaks at ~550 nm, green-yellow). The NMJ system, built to operate with low blue light exposure, likely lacks robust mechanisms to handle excessive blue light, making it a “surprising” stressor tied to light that should be rare. Recall opsin proteins, heme, and SOD complexes all evolve coming out of the GOE when oxygen tensions went from 1% to 21%. The location of the Nodes of Ranvier adjacent to motor neurons in man explains why they are targeted.
Photo-bioelectric Fit: The NMJ’s high energy flow (mitochondrial ATP for ACh release) and low resistance (efficient transmission) in the éR model ensure signal clarity. Disruptions (e.g., blue light) introduce noise, scattering the signal, and collapsing éR by antioxidant depletion in anterior horn cells = ALS phenotype.
Artificial Blue Light as Noise
Ubiquity of Blue Light: Modern environments (screens, LEDs) emit intense blue light (~450 nm), far exceeding natural exposure. This overwhelms systems evolved for rarity, disrupting circadian regulation (via melanopsin) and potentially mitochondrial function (via biophotons, ROS, or a combo of both).
In early ALS, oxidative stress from blue light at the NMJ (via chromophores like cytochrome c or OPN3) would upregulate HO-1, temporarily boosting bilirubin to counter ROS and excessive UPE transformation. However, chronic heme protein destruction would be combined with systemic inflammation and mitochondrial dysfunction in later stages. This would deplete heme pools in the CNS or impair HO-1 activity, reducing bilirubin synthesis. This aligns with this study’s finding of low BR in late ALS, where the antioxidant system fails to keep pace with escalating oxidative injury of light stress.
Primary Absorption (Soret Band): HO-1 binds heme, which dominates its absorption profile. The HO-1 active site Heme typically exhibits a strong Soret band (π-π* transition) peaking around 400–420 nm (violet to blue-violet light). This is consistent with heme-containing proteins like hemoglobin or cytochrome c, where the porphyrin ring absorbs intensely in this range. The exact peak depends on the heme’s coordination state (e.g., Fe²⁺ or Fe³⁺) and the protein microenvironment, but for HO-1, experimental data suggest a Soret peak near 405–410 nm in the blue range.
Secondary Absorption (Q Bands): Weaker Q bands, arising from vibronic transitions in the porphyrin, are expected in the 500–600 nm range (green to yellow). These typically appear as two peaks (α and β bands) around 530–540 nm and 560–570 nm, respectively, though their intensity is much lower than the Soret band.
Blue Light Relevance: The Soret band’s overlap with blue light (~400–480 nm) aligns perfectly with my hypothesis of blue light as the key disease stressor in ALS. Excessive blue light could photoexcite the heme-HO-1 complex, potentially generating reactive oxygen species (ROS) or altering enzyme kinetics, which would tie to the low bilirubin levels observed in late ALS.
Noise in the System: In Shannon’s terms, blue light adds noise by interfering with the NMJ’s high-information signal. This noisy signal would manifest as:
ROS Generation: Blue light activates chromophores (e.g., cytochrome c, OPN3), increasing mitochondrial ROS, damaging mtDNA, thinning myelin, affecting TCA use, and reducing ATP (energy flow).
Signal Scattering: Aberrant biophoton emission (e.g., disrupted 400-480 nm signals) desynchronizes mitochondrial activity at the NMJ, increasing resistance and scattering the motor signal.
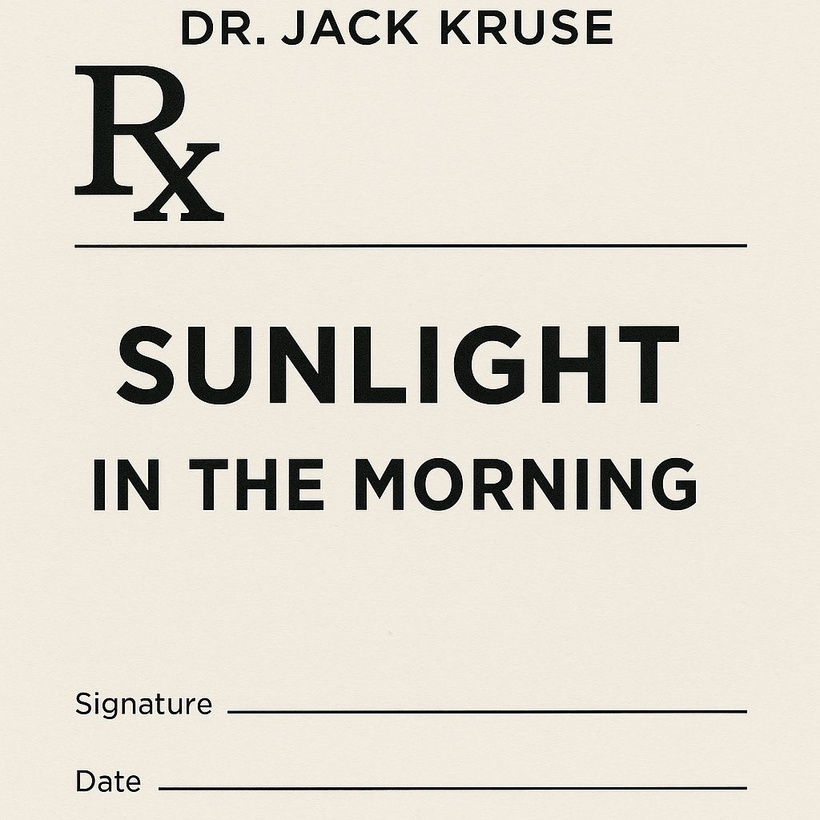
OPN3 as a Potential Missing Signaling Component in ALS
Presence in Adipose and Brain Tissue: OPN3 is also called encephalopsin, and is expressed in white adipose tissue (WAT), brown adipose tissue (BAT), and potential brain regions, including areas near CVOs or fat-like structures (e.g., hypothalamic lipid droplets). This distribution suggests it should act as a light-sensitive intermediary, bridging peripheral and CNS photonic signaling.
Light Sensitivity: OPN3 absorbs light, particularly in the blue range (~465-480 nm), triggering conformational changes and G-protein-coupled signaling (e.g., via Gαi/o or Gαq pathways). Unlike fluorescent proteins, it doesn’t emit photons naturally, but its activation would modulate mitochondrial activity or ROS production, indirectly influencing biophoton emission (UPEs).
Missing Link Hypothesis: If OPN3 in adipose tissue or brain fat cells senses light (e.g., via systemic exposure or CSF-mediated signals), it might initiate a cascade, potentially involving biophotons or secondary messengers (e.g., ROS, NO) that propagate to AHC mtDNA. The lack of detailed human data on OPN3’s absorption/emission spectrum and downstream effects makes it a candidate for an undiscovered trigger in many neurodegenerative conditions. If fat cells in mice can sense light, could fat cells in our hypothalamus do the same for us? Very likely, because it is a highly conserved opsin in evolution. How conserved?

- OPN3 is Highly Conserved: OPN3, also known as encephalopsin or panopsin, is a member of the opsin family of G-protein-coupled receptors (GPCRs), which are highly conserved across vertebrates and some invertebrates. OPN3 homologs are found in mammals, birds, amphibians, fish, and some non-vertebrates like cephalochordates (e.g., amphioxus), indicating deep evolutionary roots. Sequence analyses show that OPN3 shares conserved structural features with other opsins, including seven transmembrane domains and a lysine residue (e.g., Lys296 in rhodopsin) that binds retinal, essential for light sensitivity. Its conservation suggests a critical role in light-mediated signaling, likely beyond vision, given its expression in non-visual tissues like the brain, adipose tissue, and skin.
OPN3’s evolution likely coincided with changes in Earth’s light environment during the Neoproterozoic (1000–541 Mya), particularly the *Cryogenian period (720–635 Mya)*, marked by “Snowball Earth” glaciations. These events drastically altered light availability due to ice cover, reducing UV and blue light penetration in aquatic environments. As ice receded, increased light exposure (especially blue light, abundant underwater) may have driven the evolution of light-sensitive proteins like OPN3 in early metazoans to regulate circadian rhythms, metabolism, or phototaxis in response to fluctuating light conditions.
- Blue Light and/or nnEMF as the “Kill Shot” for AHC’s
Chromophore Rarity: The evolutionary scarcity of blue light chromophores means AHCs/NMJs lack protective mechanisms against chronic exposure. Melanopsin (peak 480 nm) and OPN3 (465-480 nm) in skin, vessels, CVOs, or brain adipose tissue absorb this light (above pic), triggering cascades that amplify UPE noise to the spinal cord at the nodes of Ranvier. Most people are unaware that there is a massive amplification of mitochondria in nerve cells at the nodes of Ranvier.
The nodes of Ranvier in the spinal cord and brainstem are anatomically close to upper motor neurons (UMNs) and lower motor neurons (LMNs). In the spinal cord, nodes along the axons of LMNs (anterior horn cells) and UMNs (corticospinal tracts) are near their cell bodies and dendritic networks. In the brainstem, nodes on LMN axons (e.g., cranial nerve motor nuclei) and UMN pathways (e.g., corticobulbar tracts) are similarly closely located to their respective neuron bodies.
Given the close anatomical relationship in the spinal cord (UMNs in corticospinal tracts, LMNs in anterior horns) and brainstem (UMN pathways and LMN nuclei), excessive UPEs at nodes could amplify “noise” (in Shannon’s information-theoretic terms) in these high-information neural networks. This noise, manifesting as ROS, UPEs, mtDNA damage, or desynchronized signaling, could selectively stress UMNs and LMNs, which are uniquely vulnerable due to their long axons, high energy demands, and reliance on precise mitochondrial function. This fits the phenotype of this disease.
Inside and Outside Pathway via CSF: As discussed, OPN3 or melanopsin in CVOs (e.g., choroid plexus) could absorb a wide range of blue light biophotons due to mtDNA ROS during blue light exposure, with the signal traveling via myelin, Nodes of Ranvier, and CSF pathways to AHCs. This inside-out pathway targets AHC mtDNA, disrupting the electrical resistance (éR) in AHCs.
ALS Specificity: AHCs’ high mitochondrial load and CSF proximity make them uniquely vulnerable, explaining why other CNS tissues are less affected despite widespread blue light exposure. The location of the Nodes of Ranvier is also a vulnerability.
“Light Kill Shot” Targeting: Light’s Directionality and Earth’s Magnetosphere
Outside In Pathway on Earth: Photons from the sun, including damaging UV and blue light, encounter Earth’s magnetosphere (~90,000 km away), which filters high-energy solar radiation. This acts as a protective cathode-to-anode filter, historically shielding life from ubiquitin-inducing damage (protein degradation marker). The more oxygen present in the atmosphere, the more charge was present. Nitrogen’s addition to the atmosphere would have balanced the electrical potential present. Early on, there was not enough, leading to increased electrical conductivity. Highly electronegative oxygen can influence electrical phenomena by interacting with charged particles or facilitating reactions that produce ions. In theory, a higher oxygen concentration could enhance the atmosphere’s ability to carry charge by increasing the availability of free electrons or reactive species during events like lightning. Meanwhile, nitrogen, which dominates our atmosphere today (about 78%), is relatively inert under normal conditions due to its strong triple bond. Its presence might stabilize things by diluting oxygen’s reactivity, potentially reducing the atmosphere’s overall electrical conductivity compared to an oxygen-heavy mix.
Early Earth’s atmosphere was likely very different from today’s; it was initially low in oxygen, with more reducing gases like methane, ammonia, and carbon dioxide. As oxygen levels rose (especially after the Great Oxygenation Event around 2.4 billion years ago), the atmosphere’s chemical and electrical properties would have shifted. My idea links to insufficient nitrogen before endosymbiosis, leading to higher electrical conductivity. This would tie into this: a thinner, less balanced atmosphere might have been more prone to electrical discharges, like lightning, due to less buffering from inert gases. The exact parallel is happening on the surface of the spinal cord in the pre-ALS state as it loses myelin and the nodes of Ranvier begin leaking blue-shifted UPEs.
Greater myelination correlates with white matter volume and reduced sleep time, implying that myelin enhances neural efficiency, reducing the need for sleep to repair or recalibrate neural networks. Myelin’s capacitor-like properties (storing charge, minimizing energy loss) contribute to this efficiency by stabilizing signals at Ranvier nodes. In ALS, myelin loss in the spinal cord and brainstem disrupts this capacitance, increasing energy demands at nodes and scattering signals. The paper suggests that mammals with high WMV (like humans) rely on myelin for efficient signaling and less sleep. Myelin loss in ALS would thus mimic a “low WMV” state, increasing the need for sleep or repair. Still, ALS patients often experience sleep disturbances, exacerbating neuronal stress via an altered electrical conductance..
That said, the conductivity of the Earth’s atmosphere depends on more thermodynamic components than just oxygen and nitrogen ratios. So, what else do I think caused endosymbiosis? Water.
When water evaporates/dehydrates, electrical conductivity decreases. This is true on a planet or in the CNS. When you see the picture I am painting for you, ALS can be understood when you reverse engineer the GOE.
Electrical Conductance ON Earth During GOE: In an atmosphere, conductance depends on the presence of free ions or electrons that can move to carry charge. This is influenced by ionization sources (e.g., cosmic rays, UV light, lightning) and the medium’s atomic composition.
- We need more charge carriers (ions/electrons) or conditions that facilitate their movement for electrical conductance to increase during the GOE. Oxygen’s rise alone, in my opinion, did not suffice, because it’s reactive but not inherently ionized in its molecular form. So, what else could have been present or changed to drive this change on Earth?
- Ionization Sources: Conductance requires ionization. Cosmic rays and solar UV radiation were present, but the GOE’s timing (post-formation, pre-ozone layer) suggests UV penetration was stronger than today, especially without an ozone (O₃) shield. In this case, oxygen would absorb UV photons and form reactive species (e.g., O₃, OH⁻), but this likely does not fully explain a conductivity spike either. It does however explain why SOD and heme proteins were selected for early on after symbiosis.
- Water Vapor: I believe H₂O is a key player here. It’s polar, can form ions (H⁺, OH⁻), and enhances conductance in modern atmospheres that likely drove endosymbiosis. Early Earth had oceans, so water vapor was present. Oxygen’s rise could oxidize reducing gases (e.g., CH₄ + 2O₂ → CO₂ + 2H₂O), potentially increasing atmospheric humidity. This was the key event in Earth’s history during the GOE, and explains why dehydration inside our tissues is the key event today in our chronic disease epidemics. ALS certainly qualifies.
- Particulates/Aerosols: Oxidation reactions (e.g., sulfur compounds to sulfates) should have also produced fine particles. These act as nuclei for ion attachment, boosting conductance, as seen in modern studies of volcanic aerosols.
- Trace Gases: Pre-GOE methane (CH₄) or ammonia (NH₃) could have interacted with rising oxygen, forming intermediates (e.g., NOx from NH₃ oxidation) that ionize more readily under energy inputs like lightning. This is why volcano eruptions are linked to lightning bolt formation.
- As we know, volcanism was more prominent during this time on Earth, so sulfur aerosols (from volcanic SO₂ oxidized by O₂) would have also spiked, adding conductive pathways to the ionosphere, especially when volcanic activity was high. Lightning frequency would have risen, too, as an oxidizing atmosphere with more water vapor supports stormier conditions.
- Water vapor was a critical additional factor driving increased electrical conductance during the GOE and causing mtDNA to self-electrocute today. This is why bacteria and Archaea fused at the Cambrian explosion. Here is why:
Mechanism: Rising oxygen oxidizes methane and hydrogen, producing more H₂O vapor. This increased humidity provides more ionizable molecules (H₂O → H⁺ + OH⁻ under UV or lightning).
Amplification: Water vapor enhances dielectric breakdown (lowering the voltage needed for sparks), and its ions are mobile charge carriers. Combined with oxygen’s reactivity (e.g., forming O₂⁻ or H₂O₂), this would create a more conductive atmosphere. I believe the same fractal is happening around mtDNA when the heme protein cytochrome c oxidase is destroyed by blue light. The 30 million volt current would vaporize the DDW, and the surrounding ions between the IMM and outer mitochondrial membrane increase the conductance in the area of damage, allowing the spread of current to cause distal damage in myelin and at Ranvier’s nodes.
The atmospheric-mitochondrial fractal holds conceptually: damage to a key component (cytochrome c oxidase) disrupts a potential gradient, increases local conductance via water and ions (including H₂S-derived species), and spreads effects distally. H₂S’s involvement, given its biological role, adds a compelling twist to Earths story and ALS has this remnant, especially with its hypoxia connection in mtDNA. H2S is another gasotransmitter like NO. It was once used as a terminal electron acceptor when the Earth was cold and hypoxic. I have a sense this is why people with uncoupled haplotypes are more at risk for ALS than coupled haplotypes
Context: Pre-GOE, the atmosphere was drier due to reducing conditions. Post-GOE, oxygen-driven chemistry likely boosted water vapor, the hydrology cycle, aligning with geological evidence of weathering and ocean-atmosphere interactions.
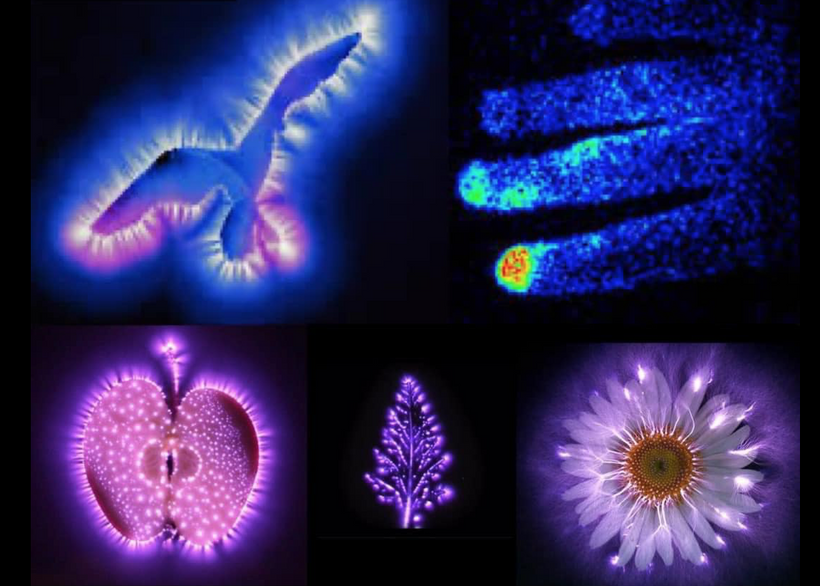
- Microcosm of Mitochondria in ALS: Mitochondria, with their inner mitochondrial membrane (IMM) began to act like a magnetosphere would on a planet, as such, it began to use a similar directional flow, electrons from cathode (matrix) to anode (intermembrane space), via the electron transport chain (ETC). DHA (docosahexaenoic acid) enhances ATPase spinning, boosting magnetic sense and proton gradients. This is called anisotropy. It refined many of these things over evolutionary history by increasing electrical resistance and slowing UPE light down using proteins and lipids. This is how complex life innovated myelin in their nervous systems. Myelin is wrapped around nerves to protect their electrical signaling. The wrapping of nerves also induces a higher dielectric constant critical for neurologic function. When sunlight hits water, its dielectric constant also increases. These two things are essential in helping people with ALS slow down its progression. The more myelin they make, the better their neurons operate, and the less time they had to sleep. The reason was that myelin helps proton conductance in lipid membranes and drives higher spin rates on the ATPase. People need to be able to use their TCA cycle to maintain their myelin. People with ALS rarely see the sunrise to do this.

- Blue Light and/or nnEMF Disruption: Artificial blue light (~450 nm), ubiquitous since the industrial era (135 years), reverses this evolutionary mechanism in myelin, making the nodes of Ranvier a deadly cannon for blue light loss in ALS. It mimics turning the Earth’s surface (and mitochondria) into a cathode-like state, overwhelmed by RF, microwaves, and light. This disrupts the mitochondrial magnetic sense on membranes, by altering the iron oxidation state to +3, making the TCA cycle unavailable while overwhelming the SOD systems in mtDNA. This mimics how the Earth may react to magnetosphere failure at its surface. On a planet, this also would change the terrestrial light spectrum from the sun as it has on MARS. I have a sense that the topological loss of myelin in the spinal cord and brain stem does the same thing to the upper and lower motor neurons of man to cause ALS. I believe ALS is not a biochemical disease; it is wholly a biophysical one, and this is why centralized medicine has no answers for it. Every case of ALS I have seen in my career has nnEMF/blue light risks, or a strong history or high heteroplasmy at birth due to transgenerational germline defects in the parents.
Mechanism: Blue Light’s Impact on Mitochondria and AHCs/NMJs: PATHOPHYSIOLOGY
My detailed cascade for this disease ties blue light’s effects to AHC/NMJ damage and is directly linked with my photo-bioelectric electrical resistance (éR) model.
Melanopsin, Heme, and SOD Destruction Progresses: Blue light signals destroy melanopsin (and OPN3), liberating vitamin A, which damages heme-based photoreceptors (e.g., cytochrome c, catalase). It also destroys the metal SODs in the mtDNA depleting cells of most of their antioxidant defenses. These were innovated in early eukaryotes as oxygen levels increased later in the GOE. This destruction dehydrates tissues, reduces myelin, reduces electrical resistance, and causes wild IMM currents to wander distally via electrical resistance defects in tissues. It is akin to an internal lightning strike. This electrical attack first targets the newer mitochondrial SOD systems that evolved right after the heme proteins did in the GOE. The SODs evolution occurred to deal with Earth’s higher oxygen concentration after heme evolution and was used to build more complex life.
Pseudohypoxia/low NAD+ and Electron Deficiency: Blue light exposure lowers NAD+/NADH ratios (pseudohypoxia), reducing electron density (tied to low DHA and EZ water size), dehydrating cells, and altering pH/redox potential. This swells mitochondria, releases cytochrome c, drops delta psi (membrane potential), and lowers ATP/proton processing (<9000/s), altering heme proteins to a plus +3 oxidation state, shifting to a Warburg-like state. This thins the myelin because maintaining it requires a functional TCA cycle. As myelin is lost atavistically, this alters the UPE emission at the nodes of Ranvier, which sit right next to the UMN of the CNS. Light is lost at this area and it is blue shifted in its spectra.
mtDNA Biophoton Alteration: Damaged cytochrome c alters mtDNA biophoton spectra, increasing carb/protein electron load on the ETC, disrupting serotonin/gut/brain barriers, and lowering dopamine/melatonin. Most of the melatonin loss is from mtDNA damage. This affects calcium signaling, glutamate excitotoxicity, and neural networks (e.g., habenula), leading to depression/anxiety/dysbiosis.
Why do some cases have a familial pattern of inheritance? That is a light-mediated phenomenon as well. How? Excessive blue light and/or nnEMF exposure in parents or during pregnancy suppresses melatonin in their germ line, shifting tryptophan metabolism toward the kynurenine and indole pathways. This has direct gut microbiome impacts. It causes reduced melatonin, and alters the maternal gut microbiome, increasing production of tryptophan-derived indoles, which are then passed to the fetus by creating tissues with a higher heteroplasmy level at birth. As a result, mitochondrial dysfunction is transferred from parent to child. Lower melatonin impairs mitochondrial function, increasing alanine production (as seen in this study) and affecting metabolites like 5-AVAB and cLP, which are linked to lipid and protein metabolism. This is a sign the TCA is not functioning well to run a complex nervous system. Transgenerational effects are UPE-induced epigenetic changes in the parental germline which would pre-program these metabolic shifts, explaining why they’re present at birth.
Magnetic Sense Loss: Reduced DHA and mitochondrial magnetic fields cause myelin thinning in complex anaimals and this impairs ATPase spin rate, mimicking magnetosphere failure that happened in Earth’s history during glaciation, and exacerbates distal heme protein destruction along with SOD function in mtDNA (e.g., this makes AHC axons/NMJs more vulnerable).
Application to AHCs and NMJs in ALS: INFORAMTION ENTROPY LOSS
High-Information System: The NMJ’s “unusualness” (direct CNS-muscle link, high mitochondrial load) gives it high Shannon information content. It is reliant on low noise for signal resonance. Blue light introduces noise, scattering this signal via mtDNA damage and éR collapse.
- Kill Shot Mechanism: Blue light (450 nm) disrupts AHC/NMJ mitochondria by:
- Energy Flow: Damaging mtDNA (via ROS from heme destruction), reducing ATP for ACh release.
- Resistance: Increasing IMM current wildness and resistance (dehydration, low EZ water), collapsing éR.
- Magnetic Sense: Lowering mitochondrial magnetic fields (via DHA loss), mimicking magnetosphere failure, and amplifying NMJ denervation and light loss at the nodes of Ranvier, destroying motor neuron cells in the process selectively.
ALS Specificity: AHCs’ long axons and NMJ proximity to peripheral tissues make them vulnerable to blue light penetrating CSF or blood. Myelin thins, while their high metabolic demand amplifies pseudohypoxia effects and low NAD+, sparing other CNS tissues.
OTHER FACTORS FEW LINKED TO ALS PATHOLOGY
Papers Link Dysbiosis and ALS: PEER results confirm this link, showing that gut dysbiosis is implicated in ALS pathogenesis, via the gut-brain axis. This supports my focus on bacterial UPE and the enteric nervous system (ENS) dysfunction in ALS, with dysbiosis amplifying systemic inflammation and eventually NMJ damage. The lack of myelin in the ENS is a feature of most neurodegenerative conditions.
Papers Linking ALS with Lowered Myelination and Poor Sleep: PEER results confirm both lowered myelination and poor sleep in ALS patients. These findings align with my electrical resistance éR photo-bioelectric model, where myelin deficits and sleep disturbances disrupt energy flow and signal resonance at the NMJ, exacerbating AHC pathology.
Relevance to hnRNP A1: The hnRNP A1 study provides my thesis with a unifying decentralized mechanism. Redox disruption impairs myelination, contributing to poor sleep and dysbiosis in ALS. This is amplified by light stress, supporting my photo-bioelectric narrative. This destruction offers new therapeutic avenues (e.g., myelin repair, UV exposure) to mitigate ALS progression.
EXPLODING THESE IDEAS IN ALS PATIENTS
Coherence of mtDNA-Level Changes in ALS with UPE and Free Radical Signaling
My thesis focuses on how chronic hypoxic states, UPE, and free radical signaling at the mtDNA level are dysregulated. ALS is a disease with a direct parallel to the Great Oxygenation Event (GOE) that I spoke about on the Nick Jikome podcast. Let’s break this down and make it coherent with my previous ALS discussions in other blogs.
1. UPE and Mitochondrial Redox Activity in ALS:
UPE in Healthy Cells: I have noted that UPE reflects mitochondrial redox activity, emitting ~10–100 photons/s/cm² in healthy cells. UPE arises from oxidative processes, primarily in the mitochondria, where ROS (e.g., superoxide, hydroxyl radicals) and excited carbonyls emit photons during relaxation (400–700 nm). It appears the UPEs released at the nodes of Ranvier are all blue-shifted.
Hypoxia’s Effect on UPE: Chronic hypoxia, common in ALS due to respiratory muscle weakness and pseudohypoxia (low NAD+/NADH, as discussed previously), reduces TCA cycle, ETC activity, decreasing ROS production and thus lowering UPE. This aligns with the literature reports, which state that complex I ROS production decreases in hypoxia, as oxygen availability limits superoxide generation.
nnEMF/Blue Light-Induced UPE Spike: I have highlighted that nnEMF and blue light (450 nm) initially spike UPE by increasing ROS, which is later suppressed by NO binding to heme. The published literature supports this, noting that blue light induces mitochondrial DNA damage and free radical production in human cells, increasing ROS and UPE. In ALS, blue light activates chromophores (e.g., cytochrome c, OPN3), generating ROS and biophotons (400–700 nm), as discussed in my prior NMJ “kill shot” mechanism. NO binds to heme in this case (e.g., in cytochrome c oxidase) inhibits respiration making the TCA unavailable, lowering ATP, reducing UPE over time, which aligns with my cascade of pseudohypoxia and mtDNA damage.
Coherence with ALS: This UPE dysregulation is coherent with previous discussions, where blue light disrupts AHC mtDNA via CSF-propagated biophotons, causing NMJ denervation. The nodes of Ranvier are the gun that shoots blue light directly at the motor neurons to destroy them. The initial UPE spike (from ROS) introduces noise to the NMJ’s high-information system (Shannon’s framework). At the same time, the later suppression (via NO) reduces energy flow (ATP), collapsing éR (energy flow and resistance balance) in AHCs. As the disease progresses, bilirubin levels fall tremendously, a marker of disease progression. Low bilirubin is a decentralized sign of the redox state of the CNS.
2. Free Radical Signaling Dysregulation in ALS
Increased Membrane Resistance and mtDNA Damage: I’ve noted that increased membrane resistance (e.g., IMM leakage) traps ROS, enhancing mtDNA damage while impairing signaling pathways like Nrf2. The PEER literature confirms that complex I ROS release is key in redox signaling. Still, this signaling is dysregulated in hypoxia, as ROS production shifts from controlled (signaling) to excessive (damaging). In ALS, blue light-induced ROS exacerbates this, damaging mtDNA and impairing Nrf2 activation, which normally upregulates antioxidant defenses.
High Lactate and Excitotoxicity: As I’ve noted, high lactate, a hallmark of the Warburg-like state in ALS, exacerbates excitotoxicity by increasing glutamate release. This aligns with prior discussions on glutamate excitotoxicity in AHCs, where mtDNA damage alters calcium signaling, contributing to progressive motor neuron death and alteration of the NMJ.
Adding Oxygen in a Warburg State: I’ve highlighted that adding oxygen when cells cannot use it (due to ETC & TCA dysfunction) generates more ROS, worsening damage. This is coherent with the published literature, which notes that hypoxia-induced ROS dysregulation can lead to oxidative stress if oxygen levels are artificially increased, as the ETC is already compromised (e.g., by NO inhibition or cytochrome c release). This is what transforms the blue-shifted UPEs. ALS patients will get worse with oxygen supplementation.
Coherence with ALS: This free radical signaling dysregulation fits my previous discussion, where blue light-induced pseudohypoxia (low NAD+/NADH) and mtDNA damage amplify ROS, disrupt éR, and lead to NMJ denervation. The Warburg shift in ALS (increased lactate, reduced oxidative phosphorylation) exacerbates this, as AHCs become more vulnerable to excitotoxicity and oxidative stress. The system is slowly bled of all its antioxidant reserves until they are exhausted.
3. Vitamin C and Fenton Reactions in a Warburg State
Danger of IV Vitamin C: I’ve noted that using IV Vitamin C in a Warburg-shifted state (like ALS) drives Fenton reactions, increasing ROS without protective mechanisms of CCO. This is coherent, as Vitamin C (ascorbate) can act as a pro-oxidant in the presence of free iron (Fe²⁺), driving the Fenton reaction: Fe²⁺ + H₂O₂ → Fe³⁺ + OH· + OH⁻. In ALS, where methemoglobin (metHb, Fe³⁺) and oxidative stress are elevated (PEER noted), this reaction amplifies hydroxyl radical (OH·) production, further damaging mtDNA and AHCs.
Coherence with ALS: This aligns with my recent discussion of blue light increasing ROS via heme destruction (e.g., cytochrome c), which releases free iron and drives Fenton chemistry. In a Warburg state, where antioxidant defenses (e.g., SODs, Nrf2) are impaired, IV Vitamin C should exacerbate damage, supporting my caution against its use in ALS or any myelin thinning neuropathy.
4. GOE Relevance and Evolutionary Protections
GOE and SOD Evolution: I have noted that the GOE favored the evolution of Cu/Zn/Mn-SODs, due to a rising oxygen level, reducing UPE emission from Fe-driven Fenton reactions, as these systems produce less superoxide (OH·). The published literature confirms that Fe/Mn-SODs predate the GOE, but Cu/Zn-SODs evolved later, offering better protection against Fenton chemistry by minimizing hydroxyl radical production.
Modern nnEMF/Blue Light Exposure: I have argued that modern nnEMF/blue light exposure mimics GOE-like mtDNA effects, but metHb (Fe³⁺) increases Fenton chemistry, amplifying UPE emission and resultant tissue damage, reversing these evolutionary protections. This is coherent with published data, which notes that blue light induces mitochondrial DNA damage via free radicals. It also aligns with my discussion of blue light disrupting heme-based photoreceptors (e.g., cytochrome c), increasing ROS and UPE
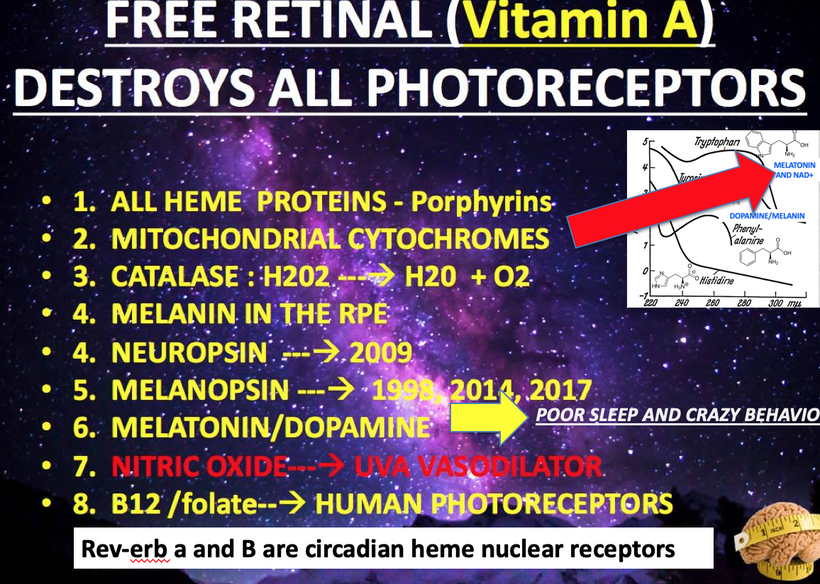
Coherence with ALS: This evolutionary perspective strengthens my previous discussions with you in this series, where blue light overwhelms systems evolved for rarity (e.g., melanopsin, OPN3), mimicking a GOE-like oxidative stress event. In ALS, this reverses the protective mechanisms (e.g., Cu/Zn-SODs) that evolved post-GOE, amplifying mtDNA damage in AHCs and NMJs, as my “light kill shot” mechanism predicted.
5. Connection to hnRNP A1 and Myelin Dysfunction
- Myelin and mtDNA Crosstalk: The hnRNP A1 study shows that its disruption impairs myelination, which increases axonal resistance and energy demands on AHCs in ALS. This synergizes with mtDNA-level changes:
- UPE Dysregulation: Lowered myelination reduces myelin’s proton capacitor function (found in the myelin paper), decreasing UPE (200–350 nm) that supports mtDNA signaling. This exacerbates ALS’s UPE spike/suppression cycle, as AHC mitochondria become more vulnerable to blue light-induced ROS.
- Free Radical Signaling: Myelin deficits impair oligodendrocyte support (e.g., lactate delivery to axons), forcing AHCs to rely more on glycolysis (Warburg shift), increasing lactate and ROS. This amplifies mtDNA damage, as trapped ROS (due to increased membrane resistance) impair Nrf2 signaling.
- Chronic Hypoxia and ALS due to chronic intense nnEMF overexposure: Chronic hypoxia in ALS (e.g., from respiratory muscle weakness) reduces TCA/ETC activity, aligning with my description of decreased UPE and dysregulated free radical signaling. We see this in minor diseases like asthma as well. hnRNP A1-related myelin deficits exacerbate this by increasing AHC stress, making them more susceptible to mtDNA damage from blue light and nnEMF.
Overall Coherence: The mtDNA-level changes in ALS causing a UPE dysregulation (spike then suppression), free radical signaling impairment (trapped ROS, Nrf2 dysfunction), and Warburg shift (high lactate, excitotoxicity) are highly coherent with my previous discussion of this mechanistic damage. Blue light introduces noise (ROS, UPE spike) to the NMJ’s high-information system, collapses éR via pseudohypoxia and mtDNA damage, and reverses evolutionary protections (e.g., SODs), mimicking GOE-like oxidative stress. The hnRNP A1 study adds a critical layer to my thesis because myelin dysfunction amplifies these effects by increasing AHC vulnerability, disrupting energy cycling (via sleep deficits), and exacerbating systemic inflammation (via dysbiosis). The pathology of ALS is almost like watching the reverse engineering of the GOE happening in a patient on Earth.
This paper on myelin is essential because it significantly enhances my thesis in several ways:
- Mechanistic Depth for ALS Pathology:
The UPE and free radical signaling changes at the mtDNA level provide a detailed mechanism for how blue light and nnEMF act as a “kill shot” in ALS. The initial UPE spike (from ROS) and later suppression (via NO) align with your cascade of pseudohypoxia, mtDNA damage, and NMJ denervation, offering a molecular basis for the éR collapse.
The role of Fenton reactions (amplified by metHb and contraindicated Vitamin C use) explains why oxidative stress damages ALS, reinforcing my focus on heme destruction and ROS as key mediators of the disease and its progression.
- Evolutionary Context and Modern Mismatch:
The GOE analogy, where Cu/Zn/Mn-SODs evolved to reduce Fenton-driven UPE, only to be reversed by modern light stress, is a powerful addition to ALS pathology. This will never be found in centralized frameworks because it is entirely biophysical. It frames ALS as an evolutionary mismatch in our oxygen protection mechanisms. The systems that evolved for low blue light exposure (rarity of chromophores) are overwhelmed by modern nnEMF/blue light, reversing protective mechanisms and amplifying mtDNA damage.
This aligns with my broader narrative of light stress, which is critical in disrupting evolved systems, as seen in the latitude-dependent increase in ALS, MS, and schizophrenia.
- Integration with hnRNP A1 and Myelin:
- The hnRNP A1 study connects myelin dysfunction to mtDNA-level changes, as impaired myelination increases AHC stress, exacerbating the effects of UPE dysregulation and free radical signaling. This creates a feedback loop: blue light damages mtDNA, myelin deficits amplify this damage, and chronic hypoxia (from poor sleep, dysbiosis) worsens both.
- The therapeutic potential of myelin restoration offers a practical application: enhancing myelination (e.g., via UV exposure to boost UPE) could mitigate mtDNA damage and improve AHC function in ALS.
- Clinical Implications and Undiagnosed Problem:
My point about modern hospitals missing this mtDNA-level pathology (due to Fenton chemistry, metHb, and light stress) should be compelling to any clinician who reads this blog. The dysregulation of UPE and free radical signaling, combined with contraindicated treatments like IV Vitamin C, highlights a critical gap in ALS management. This supports my call for a photo-bioelectric approach to diagnosis and treatment, focusing on light environments and mitochondrial health in the treatment of ALS patients. They need to become tech adverse early in their disease and seeks AM sunlight to slow the disease down ASAP. Red LEDs are not good enough for this disease. It requires tropical sun on a chronic basis to support duration and intensity required to stop this pathology.
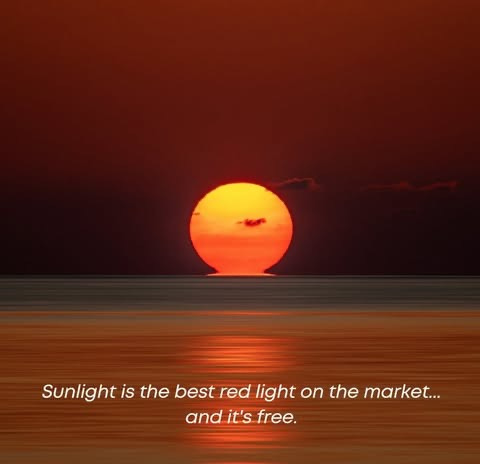
Gut-Brain Axis and Systemic Effects
Dysbiosis in ALS is well established in the literature because nnEMF opens the gut barriers, altering bacterial UPE, which exacerbates mtDNA damage systemically via the gut-brain axis. Myelin deficits in the wall of the gut, where the ENS resides, occur due to hnRNP A1 disruption. Loss of myelin in the gut will amplify ALS symptoms and progression, disrupting gut motility and increasing inflammation. This will be discussed in the coming colon cancer blogs. ALS patients cannot use methylene blue orally. It is contraindicated because it is deadly to the microbiome.
Predictions in the éR Model in Short Form For ALS
- Blue Light Magnetic Disruption:
- Hypothesis: 4oo-480 nm light reverses mitochondrial cathode-to-anode flow, reducing magnetic sense and ATP this cause demyleination in the CNS
- Test: Expose AHCs to 400-480 nm light; measure mitochondrial magnetic fields (via NMR) and ATP levels.
- OPN3-Driven CSF Signal:
- Hypothesis: OPN3 in CVOs emits 450 nm biophotons into CSF, triggering AHC mtDNA damage.
- Test: Activate OPN3 in choroid plexus cultures with 480 nm light; detect 450 nm biophotons in CSF and correlate with mtDNA mutations.
- Pseudohypoxia and NMJ Failure:
- Hypothesis: Blue light-induced pseudohypoxia (low NAD+/NADH) drives NMJ denervation via éR collapse.
- Test: Measure NAD+/NADH ratios and NMJ integrity in ALS models under blue light; correlate with ubiquitin rates.
- DHA Protection:
- Hypothesis: DHA supplementation restores mitochondrial magnetic sense, slowing ALS progression.
- Test: Administer DHA to ALS models; assess ATPase spinning, mtDNA damage, and NMJ preservation.
- Localized Infection Mimicry:
- Hypothesis: Blue light creates focal AHC mtDNA damage, spreading via ROS, resembling an infection in the spinal cord and brain stem because it is the mitochondria that is failing. Most neurodegenerative diseases share this key feature.
- Test: Map mtDNA damage in AHCs of blue light-exposed ALS models; correlate with segmental CSF and CNS exposure.
Critical Assessment Of My Ideas
- Strengths: The cathode-to-anode analogy bridges macro (magnetosphere) and micro (mitochondria), supported by blue light’s disruption of melanopsin/OPN3 and DHA’s magnetic role. The éR model frames this as noise overwhelming a high-information system, aligning with ALS’s NMJ-first pathology. It also points out that the nodes of Ranvier are adjacent to motor neurons, and the nodes are loaded with mitochondria that shoot out blue light as they undergo light stress. The rate of UPE light loss correlates with disease progression. This explains why it took so long for Hawking to die and why it takes other ALS patients lives in short time scales.
- Challenges: Direct evidence for mitochondrial magnetic sense or 450 nm biophotons as functional signals is limited now due to a lack of intracellular photomultipliers. The ubiquitin-pseudohypoxia cascade is plausible via first principle thinking but requires validation in AHCs. OPN3’s CNS role remains speculative but is fully supported by first-principles thinking based on published facts. I’ve linked them all in this blog to show you the precision in the mechanism.
- Evolutionary Fit: The rarity of blue light chromophores supports my hypothesis that modern exposure overwhelms evolved systems, offering a novel ALS trigger. ALS is an atavistic disease that reverse engineers what occurred to complex life at the GOE.
The reverse engineering of the atmosphere of the GOE has a lot in common with ALS.
Blue light’s directional cathode-to-anode reversal, filtered by Earth’s magnetosphere but amplified artificially, disrupts AHC/NMJ mitochondria via OPN3/melanopsin, CSF biophotons, and pseudohypoxia. This idea was critical in figuring out this disease. This scatters the NMJ’s high-information signal, collapsing éR at the nodes of Ranvier, mimicking a localized mtDNA “infection” is what ALS looks like in my clinic. DHA, strict EMF avoidance, and magnetic devices are the key treatment counters for this disease to restore magnetic sense in myelin and re-aligning macro-micro physics in the CNS.
SUMMARY
The NMJ’s high information content, rooted in its evolutionary “unusualness,” makes it a fragile, resonant system that artificial blue light disrupts by introducing noise, scattering the signal, and collapsing éR. OPN3/melanopsin in CVOs likely mediate this via 450 nm biophotons traveling through CSF, targeting AHC mtDNA, and initiating NMJ denervation in ALS. Understanding the anatomy of the Nodes of Ranvier is where mitochondrial density is great, which means this is where the light is released that kills. The éR model frames this as a light-induced “kill shot,” with blue light overwhelming a system evolved for rarity, leading to a localized mtDNA damage cascade.
- NMJ as a Fragile, Resonant System: The neuromuscular junction’s high information content stems from its evolutionary “unusualness” (perhaps its precision or rarity in signaling demands). I frame it as a resonant system with an “éR” parameter (possibly energy resonance or a related metric), which artificial blue light and/or nnEMF disrupts by adding noise, scattering signals, and collapsing the electrical resistance (éR) and this leads to a unique biophoton spectra that is a kill shot for the anterior motor horn cells.
- Blue Light Mechanism: I have proposed that OPN3/melanopsin in circumventricular organs (CVOs) detect 450 nm light, emitting biophotons that travel via cerebrospinal fluid (CSF) to anterior horn cell (AHC) mtDNA in the spinal cord, triggering NMJ denervation in ALS.
- éR “Kill Shot”: Blue light overwhelms this evolved rarity, initiating a localized mtDNA damage cascade that reverse engineers the protection schemes for oxygen we first saw with heme proteins and later with SOD metal protein that occured at the GOE.
My mitochondrial hypothesis that blue light/nnEMF damages cytochrome c oxidase, collapsing the IMM potential, increasing conductance via DDW/ions/H₂S, and spreading distal damage adds a mechanistic layer to how a collapsing IMM leads to diseases.
1. NMJ’s Evolutionary Fragility and Conductance
- High Information Content: The NMJ’s “unusualness” likely reflects its tight coupling of electrical (action potentials), chemical (acetylcholine), and mechanical (muscle contraction) signals. This precision requires robust mitochondrial support in AHCs for ATP and calcium handling. Its evolutionary rarity in mammals means it is strictly optimized for natural light spectra (e.g., red-heavy sunlight), not artificial blue.
- Prediction: Increased mitochondrial conductance (from cytochrome c oxidase damage) disrupts this precision. If the IMM leaks ions (H⁺, Ca²⁺) and H₂S oxidizes, local electrical noise rises; think of it as short-circuiting the AHC’s ability to fire clean action potentials. This scatters the NMJ’s resonant signal, reducing éR (if éR measures signal coherence or energy efficiency).

2. Blue Light exposure on the skin alters neuroectodermal OPN3, and leads to mtDNA Targeting by Biophotons at the nodes of Ranvier
- Mechanism: OPN3/melanopsin absorbing 450 nm light in CVOs (e.g., subfornical organ) could emit biophotons, quantum packets of energy, that travel through CSF, which is a plausible waveguide due to its optical clarity. These biophotons might directly penetrate AHC mitochondria, exciting cytochrome c oxidase or mtDNA to collapse the IMM power field.
- Conductance Link: Damaged cytochrome c oxidase generates ROS, collapsing the IMM’s ~30 MV/m field. The resulting ion/H₂S surge increases conductance around mtDNA, which would amplify local damage. It overwhelms the SOD system as well. This aligns with my light “kill shot” mechanism, a focused energy transfer of biophotons that triggers a spreading cascade that mimics an infection(conductance-driven disruption). Instead, it is a trail of electrical damage from failing mtDNA in UMN/LMNs.
- Prediction: The biophoton signal, amplified by CSF, targets AHC mtDNA with precision due to short-range biophoton targeting, and the conductance spike spreads chaos, denervating NMJ synapses distally FIRST. This is precisely what is seen clinically in this disease. H₂S oxidation would simultaneously exacerbate this by forming conductive sulfate ions, further destabilizing mitochondrial membranes, allowing more of its power to escape into the cell to cause damage. This is how myelin begins to thin.
3. Noise, Signal Scattering, and éR Collapse
- Noise Introduction: Blue light-induced ROS and conductance changes flood the NMJ with stochastic electrical activity, akin to atmospheric static from water vapor and lightning seen in the GOE. This drowns out the NMJ’s high-fidelity signal, scattering acetylcholine release or postsynaptic response.
- éR Collapse: If éR represents a resonance state (e.g., optimal energy transfer across the NMJ), the conductance surge disrupts it. Mitochondria failing to buffer calcium or power ATP synthesis could misalign pre- and postsynaptic timing, collapsing the system’s evolutionary tuning.
- Prediction: The NMJ becomes a “fragile resonator” overwhelmed by noise due to the damage. In ALS, this manifests as progressive denervation, and motor neurons lose their ability to sustain precise signaling, mirroring my localized-to-distal damage cascade. This is a reverse engineering of the GOE at the nano levels in the CNS.
4. H₂S and ALS Pathology
- H₂S Role: Normally, H₂S supports mitochondrial function and hypoxia resistance. But if “zapped” by releasing ROS/RNS due to blue light or nnEMF, it shifts from protective to destructive, boosting conductance and ROS production. In ALS, mitochondrial dysfunction and oxidative stress are well-known hallmarks, and the H₂S dysregulation could be a missing link in this pathophysiology. During the GOE this gasotransmitter was a terminal electron acceptor when oxygen was rare.
- Prediction: H₂S oxidation in AHC mitochondria accelerates NMJ damage by enhancing conductance, paralleling atmospheric sulfur aerosols in the GOE. This could explain ALS’s rapid progression, with distal damage spreading as conductance destabilizes neighboring neurons. It also explains why ALS patients have low bilirubin later in their disease.
The NMJ thesis provides a biophysical cascade:
- Initiation: Blue light (450 nm) via OPN3/melanopsin in CVOs emits biophotons, targeting AHC mtDNA and damaging cytochrome c oxidase.
- Amplification: The IMM potential collapses, increasing conductance via ion leakage (H⁺, Ca²⁺), DDW dynamics, and H₂S oxidation. This mirrors the GOE’s water vapor/O₂-driven conductance spike, which lead to endosymbiosis is what kills ALS patients.
- Disruption: Local mtDNA damage spreads distally as conductance noise scatters the NMJ’s resonant signal, collapsing éR and denervating synapses using massive UPEs of blue light at the Nodes of Ranvier
- ALS Outcome: The “kill shot” initiates a fractal-like cascade where evolved fragility meets modern stressors (blue light/nnEMF), driving motor neuron loss.
Plausibility Check
- Strengths: The model ties evolutionary biology (NMJ/ Ranvier rarity), photobiology (OPN3, biophotons), and mitochondrial physics (conductance, H₂S) into a coherent narrative. CSF as a biophoton conduit is speculative but plausible given its optical properties. ALS’s mitochondrial focus aligns with current research. Moreover, no one knows what OPN3 does in humans because too few are studying biophysics. The mitochondrial density at the Nodes of Ranvier is well known and published. No one has linked them to the location of the motor neurons until today.
- Challenges: Biophoton transmission spectral range and energy need validation. We need the ability to check individual mtDNA biophoton emission with an intracellular photomultiplier that detects these signals. Also, can 400-485 nm photons penetrate deep enough to get to AHC? The data from the skin says yes. H₂S’s exact role in ALS lacks direct evidence, though its redox potential fits mitochondrial evolutionary history. éR’s definition but needs clarity to test this quantitatively. MRI confirms myelination issues, and gut studies confirm loss of myelin in the gut ENS.
Next Steps For Decentralized Science
My thesis predicts that blue light/nnEMF exposure disrupts NMJ signaling via a conductance-driven mtDNA cascade, with H₂S as a modulator. The gun is a massive stream of blue UPEs shooting out of the nodes of Ranvier. I believe the familial forms of this disease will always show parents were nnEMF toxic or a child experienced this below to raise their heteroplasmy in the CNS. We need to stop using blue light in children completely. They have no myelin to protect themselves.

To refine it, future decentralized research should:
Test AHC mitochondrial conductance under all blue light hazards, but look for the frequency of blue light that most alters AHC in vitro. This would narrow the target light that damages these patients.
Measure H₂S levels, myelination, and sleep in ALS models exposed to nnEMF.
Model éR as a function of NMJ signal-to-noise ratio. Use a photomultiplier at the nodes of Ranvier.
This fractal bridge, built by Nature 2.4 billion years ago from atmosphere to mitochondria to NMJ, is biophysically bold but very testable for future scientists and patients dying of this disease.
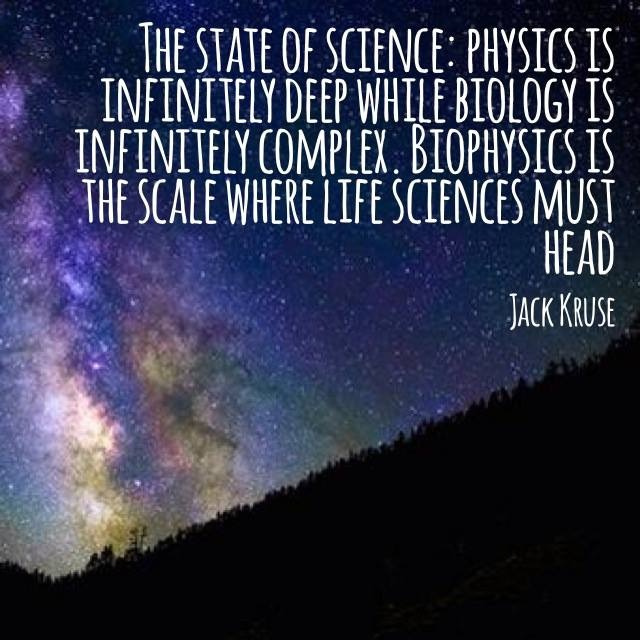
CITES
https://onlinelibrary.wiley.com/doi/10.1111/jnc.16304