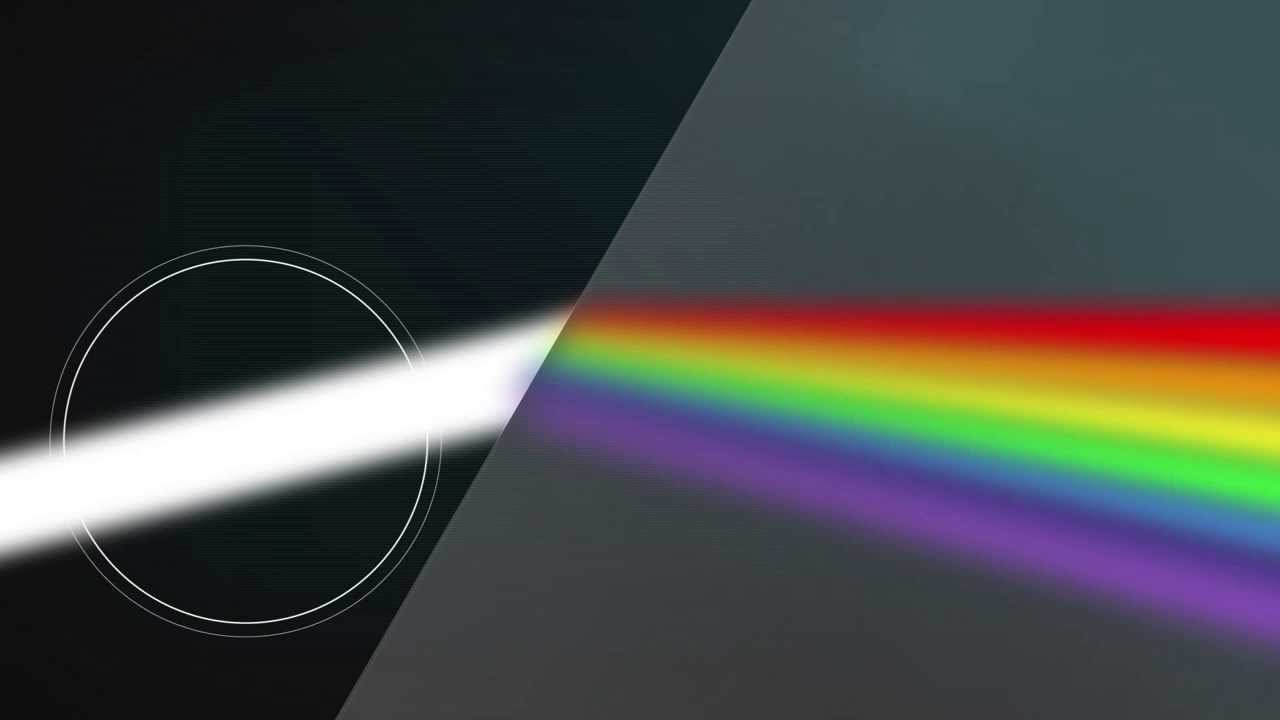
Your worldview has to be structured in principle on some specific doctrine that shapes how you function and view humanity. Embracing QED is akin to wearing steel-toed boots in a ballet-slipper world. Understanding that life is bigger than your set of challenges will help you overcome obstacles that will come your way from time to time. It’s impossible to increase the scope of our worldview when all that we are focused on is ourselves. In this way, life can be thought of as a prism. What you perceive depends on how you turn the glass. Your retina is a prism. It has photoreceptors for color vision, it has melanopsin for SCN function, it has orexin neurons, it has neuropsin, and it has Müller cells that are optical fibers (picture below) to refract the light.
Müller cells are radial glial cells spanning the entire retinal thickness. Muller cells act as astrocytes do in forming the blood-brain barrier. Müller cells have an extended funnel shape, a higher refractive index than their surrounding tissue, and are oriented along the direction of light propagation in the eye. Müller cells provide trophic and anti-oxidative support for photoreceptors and neurons and regulate the tightness of the blood-retinal barrier. I believe IR-A light has a massive effect on cytochrome c oxidase to help regenerate damaged retina.
While the exact mechanism of PBM in treating retinal regeneration is not entirely clear in the literature I have reviewed, I believe I have found the likely target. I believe that mitochondria in the Muller glial cells provide a massive source of red photons that are absorbed by the copper subunit of the terminal enzyme, cytochrome c oxidase (CCO). PBM likely affects other parts of the electron transport chains of mitochondria like the Q-cycle as well. I believe the light absorbed by CCO enhances the ability of the mitochondria to catalyze the reduction of oxygen to produce ATP more efficiently. It also augments the creation of water, which becomes a source of delocalized electrons and protons that can be used to regenerate damaged parts of the retina.
As CCO redox activity increases from PBM therapy, oxygen consumption also increases in the retina, leading to a rise in the rate of oxidative phosphorylation as well as cellular oxygen metabolism. Since neurons in the retina have an increased reliance on mitochondrial oxygen metabolism compared to most other cell types, PBM has already been shown to affect neuronal functions significantly by raising ATP production. UV light seems like it would limit regeneration by this mechanism based on the slide above.
Traumatic stress of any type can elevate glutamate to abnormally high levels in the brain/retina. Light stress increases glutamate via the activation of orexin A in the retina. A brain injury or stroke causes glutamate to flood the injured area.
Astrocytes make up the blood-brain barrier. They release vitamin C to stress to alter metabolism to help NAD+ recycle faster to create ATP. In the retina, Muller cells do the same thing for the neurons of the retina. The glial Müller cells in the retina, regulate the glutamate cycle to prevent damage in oxidative stress conditions of the photoreceptors.
PBM likely increases the uptake of glutamate, helping the Müller cells to protect the retina. They are more directly involved in the regulation of the synaptic activity in the inner retina. They help organize the chaos that light creates in the retina. Orexins seem evolved to deal with the chaos the sun creates and not any man-made light. Man-made light seems to unleash orexin A so that the PVN is chronically turned on to give us a syndrome that mimics adrenal fatigue. It should not surprise you because light stress stimulates orexin A and this activates the PVN. This also releases glutamate and an excessive amount of Vitamin C. When Vitamin C is exhausted none of the catecholamine molecules associated with adrenal fatigue can be made. This is why adrenal fatigue is not an adrenal disease. It is a disease of the eyes, orexin, and the PVN due to excessive glutamate release and excessive use of Vitamin C so that dopamine, epinephrine, and noradrenaline can’t be made.
The light is broken down into its parts to impart information to the retina. In this way, Perception is a prism, and the retina is like shot silk – the quality of information it imparts depends on the quality and quantity where the light hits.
Counterintuitively, the retina of the vertebrate eye is inverted with respect to its optical function and light must pass through several tissue layers before reaching the light-detecting photoreceptor cells. The reason for this I laid out in my Vermont 2017 talk on YouTube.
In mammals and humans, the extent of spontaneous repair after retina injury or disease is either non-existent or extremely limited (Karl and Reh, 2010). Rather than regenerate, damaged mammalian retinas commonly undergo reactive gliosis and scar formation (Bringmann et al., 2006). (See below)
This lack of a complete regenerative response to damage directly limits the treatment options for retinal-based diseases such as age-related macular degeneration or Stargardt’s disease. The use of PBM seems to help use the Muller cells to help regenerate damaged retinas. I have had quite a bit of success with this in the last three years. I read some papers on retinal damage in zebrafish and realized that Muller cells were the key to understanding how to regenerate damaged retinal tissues.
The retina forms from the central nervous system (CNS) and develops into a three-layered structure consisting of seven main types of cells and numerous other cell types identified by single-cell RNAseq (Macosko et al., 2015). The structure, function, cell types, and genes expressed in the retina are largely conserved among vertebrates, supporting the notion that information gained from models capable of spontaneous regeneration likely applies to mammals whose regenerative capacity varies. It appears humans have a lot in common with zebrafish based on my recent experience with acute macular degeneration.
The information the retina creates is stored electromagnetically in coherent domains of water in cells like the Muller glia and in the CSF that surrounds the key areas of the brain that interact with the environment. CSF is made by the choroid plexus in the brain. CSF is an ultrafiltrate of the blood.
OREXINS AS A PRISM
I’ve taught my readers about every single part of the retinal prism, but I’ve saved the orexin neurons for last. They are intercalated deep into the retina of every vertebrate on planet Earth. They are the rare neurons in the entire human brain. Scarcity creates its value to signaling when it comes to light. Light strikes things and changes them in ways our retina cannot sense. This makes light the most powerful change stimulus behind the evolutionary adaptation.
Our prism can become our prison if we don’t understand how it operates and with what light it is designed to work.
With change being an inevitable element in our lives, we have only two options. Either embrace it and live life to the fullest or be stuck in the comfort zone of a compromised life. Orexins make sure vertebrates never stay in their comfort zone of life. They create stress for us to adapt to. This response must be controlled.
Orexins are hypothalamic neuropeptides that were initially identified in the rat brain as endogenous ligands for a (previously) orphan G-protein-coupled receptor (GPCR). I spoke about them here over ten years ago. They are multitasking peptides involved in many physiological functions, including regulation of feeding behavior, wakefulness and autonomic/neuroendocrine functions, and sleep/wakefulness states in mammals. There are two isopeptides of orexin, orexin A and orexin B, which are produced from a common precursor peptide, pre-pro-orexin. UV and red light operate on these two orexins from sunlight.
The structures of orexins, as well as orexin genes, are highly conserved throughout mammalian species, suggesting strong evolutionary pressure has maintained these structures for hundreds of millions of years. In this way, orexins are like DHA they haven’t changed in hundreds of millions of years of vertebrate evolution. Note in Orexin -A below the disulfide bridges. The photosensitivity of protein-bound cysteine residues has been shown to depend on the redox state of the microenvironment (Augenstein and Ghiron 1961; Dose and Rajewsky 1962; Augenstein and Riley 1964; Dose 1964, 1967; Fiore and Dose 1965; Grist et al. 1965; Risi et al. 1967; Koudelka and Augenstein 1968)
This data set correlates with the observation by Petersen et al. made in 1999, that cystine residues (cysteines involved in disulfide bridges) have a clear preference for aromatic residues as spatial neighbors. Note that orexin has one tyrosine adjacent to its S-S bond. Why is this important? Recall that all cells release ultra-weak UV light. Going further, vertebrate eukaryotes tend to release less ultraweak UV light unless the redox state is low. When it is low eukaryotes release significantly more ultraweak UV. The excessive ultraweak UV light then disrupts the S-S of orexin A and this changes the tertiary and quaternary protein folding of orexin and alters its physiologic signaling optically. These photodynamic reactions allow for a temporally and spatially controlled and reversible modification of the surface of orexin A and, hence, can be used to produce functional interfaces that change signaling.
It has been reported that the UV illumination of Tryptophan and Tyrosine residues gives rise to delocalized electrons. UV light can also liberate hydrogen from aromatic amino acids into the hydration shell. The H-atom ejection from these amino acids was written about decades ago. (Vladimirov et al. 1970; Feitelson 1971). The role of the hydrated electron in photo-reduction of cystine in the presence of indole (melatonin is an indole) led to the proposal of a possible mechanism behind this phenomenon over 50 years ago (Grossweiner and Usui 1970). Few go back and read those papers to understand what orexins are really doing. The liberation of these electrons and protons is what changes the hydrogen bonding network to become coherent domains. Orexins are the gatekeepers that force water to take on a hexagonal form to become quantum coherent in the brain.
The connection of orexin to melatonin is more important to make.
Melatonin and dopamine are known to be critical in regenerating all human photoreceptors (see below). The problem is we do not know exactly why. Melatonin has two receptors, MT1 and MT2. The pathophysiological function of the melatonin MT1 and MT2 receptors has not yet been well-clarified. The MT1 receptor is involved in the regulation of the circadian rhythm. MT2 is not. MT2 receptors are located in the reticular thalamus which is the non-REM sleep area. Non-REM sleep disorders are also called arousal disorders. Non-REM parasomnias involve physical and verbal activity. You are not completely awake or aware during these events, are not responsive to others’ attempts to interact with you, and usually don’t remember or only partially remember the event the next day.
The MT1 receptor is in the locus ceruleus and lateral hypothalamus where REM areas exist. This is the same area where orexin neurons project to. When these orexin neurons are destroyed there we also see a REM sleep disorder develop called narcolepsy. People with narcolepsy frequently enter REM sleep rapidly with sleep onset. This usually happens within 15 minutes of falling asleep day or night. Also, muscle weakness or dream activity that is associated with REM sleep can occur during wakefulness or be absent during sleep. REM normally occurs in short periods and is characterized by a decrease in muscle tone and is associated with profound sympathetic activation (PVN), including increased heart rate, breathing, blood pressure, and temperature.
The lengths and structure of orexins suggested that orexin B is the ancestral form of the orexin neuropeptide. In mammals, orexins bind to two subtypes of GPCRs, i.e., orexin 1 receptor (OX1R) and orexin 2 receptor (OX2R).
I believe one orexin works best with UV light signaling and the other is optimized by red light. One reacts best with fast-traveling red light (orexin B) and the other with slower-traveling UV light (orexin A) in our tissues. Phylogenetically, the orexin system is present exclusively in vertebrates so this puts them around 650 million years old.
Orexin A is a 33-amino-acid peptide with an N-terminal pyroglutamyl residue, two intrachain disulfide bonds, and C-terminal amidation. Strikingly, this structure is completely conserved among all mammalian species so far identified in the literature. This means orexin A is the same in humans, gorillas, rats, mice, cows, pigs, sheep, dogs, seals, and dolphins. Very few proteins have been found to have this long-term evolutionary lineage.
Most of you have learned from me there are a lot of photoreceptors in the eye like melanopsin, melatonin, neuropsin, B12, dopamine, melanin, cytochrome c oxidase, nitric oxide, and heme proteins like catalase in RBCs etc. (see above and below)
The central retinal pathways in the retina of all mammals contain orexin neurons that project to the areas of the brain adjacent to CSF pathways. Orexin-producing neurons (orexin neurons), in the lateral hypothalamus, receive input from the forebrain structures including the extended amygdala and nucleus accumbens (NAc)—which are implicated in the processing of emotion and motivation—and send output to brain stem regions, which are implicated in the regulation of wakefulness. I told you in the cold thermogenesis series that sleep was our primordial state, and we evolved wakefulness. I believe the orexin neurons were key in this transition. Orexin made water more quantum coherent so more energy could be harvested to allow wakefulness to emerge. Orexin neurons play an important role as a link between emotional states and wakefulness states. Any acute stress activates orexins neurons, and this includes modern light stress. This is why I have believed for 15 years that adrenal fatigue is a disease of the periventricular nucleus. I believe orexins are the fuse that begins the process.
There are only 50,000–80,000 orexin-producing neurons in the human brain, located predominantly in the perifornical area and lateral hypothalamus. The hypothalamus sits adjacent to the third ventricle which is filled with CSF. CSF is 99.8% water created by mitochondria. Let’s talk about the prism that your eye clock sits in.
Light transmission via the retina interacts with the amacrine cell level in the retina which in turn activates both orexin systems. The orexin systems modulate the stress response in mammals. The orexin system was initially suggested to be primarily involved in the stimulation of food intake, based on the finding that central administration of orexin-A and -B increased food intake. In addition, they stimulate wakefulness, regulate energy expenditure, and modulate the visceral function and brown fat activation.
Many studies support that the orexin neurons regulate brown adipose tissue (BAT) activity via the sympathetic nervous system to enhance energy expenditure. This is the basis of my cold thermogenesis protocol.
Orexin promotes wakefulness. I believe inhibition of orexin function is how general anesthetics work. Right now, most anesthesiologists still have no idea how the drugs they use daily work. Studies have indicated that a major role of the orexin system is to integrate metabolic, circadian, and sleep debt influences to determine whether an animal should be asleep, or awake and active. Orexin B are proteins that absorb the slowest form of light in tissue to maximize the fidelity of the signal. Orexin A neurons strongly excite various brain nuclei with important roles in wakefulness including the dopamine, norepinephrine, histamine (all made from aromatic amino acids), and acetylcholine systems, and appear to play an important role in stabilizing wakefulness and sleep (adenosine). Adenosine is activated by IR-A light. The sleep-promoting action of adenosine can be reversed by orexin A applied to the lateral hypothalamus, by exciting glutamatergic input to orexin neurons via the action of orexin receptor 1. I believe it is the ultraweak release of UV light that disrupts the S-S bond of Orexin-A that cause sympathetic outflow to increase. This leads to REM sleep disorders = narcolepsy.
The discovery that an orexin receptor mutation causes the sleep disorder canine narcolepsy in Doberman Pinschers subsequently indicated a major role for this system in sleep regulation. Narcolepsy is a REM sleep disorder. Genetic knockout mice lacking the gene for orexin were also reported to exhibit narcolepsy.
In humans, narcolepsy is associated with a specific variant of the human leukocyte antigen (HLA) complex. A lack of either red light or UV light is how narcolepsy begins. It begins with poor light signaling in the prism of the retina. Light changes the charge density of the retina. The proof is found in genome-wide analysis studies that show that, in addition to the HLA variant, people with narcolepsy also exhibit a specific genetic mutation in the T-cell receptor alpha locus. I spoke about the HLA complex in the brain-gut series when I explained how the chimp brain evolved into the homo sapien brain using the HLA complex. I think orexins helped fuel the transition.
In conjunction, there are genetic anomalies that cause the immune system to attack and kill the critical orexin neurons. Once they are destroyed, they do not come back. Hence the absence of orexin-producing neurons in people with narcolepsy appears to be the result of an autoimmune disorder. Can we see the effect of these changes elsewhere in the brain? We do. We see it in the hydrogen bonding networks of water in cells, in the blood, and in the CSF.
All paths can be seen, through the prism of fate. Sunlight is the Windex our watery eyes require. The periventricular nucleus controls our sympathetic response by how it reacts to the orexin signal from the central retinal pathways. Moreover, the choroid plexus (CP) of the brain that creates CSF has recently been implicated in the regulation of circadian rhythmicity and therefore, it is now recognized that the functions of the CP cannot be limited to those listed in textbooks. Given the strong circadian influence over the sleep-wake cycle and orexinergic signaling, the role of the CP in modulating orexinergic signaling via circadian influence is worth further study. Furthermore, considering that the sleep-wake cycle modulates additional homeostatic processes such as amyloid-β and glymphatic clearance, the hydrogen bonding networks in the CP may also have a significant influence on the activity of these functions. People forget that the S-S bond in orexin-A induces proteins to misfold due to the effect of UV light on this bond. Brains with neurodegeneration all have low redox states and this means they will release a lot more ultra-weak UV light. This light will destroy orexin-A and lead to protein misfolding at a log rate. As this light stress develops, glutamate rises and the BBB becomes leaky. The process progresses and gets worse.
The autonomic nervous system has two main arms, sympathetic and parasympathetic. The vagus is part of the parasympathetic system that lowers the stress response in the brain stem. The periventricular nucleus is one of the key targets of the orexin neurons. Stressor activity increases chronically when the sympathetic system is constantly turned on. The sympathetic system outflow of the stress stimulus begins in the paraventricular nucleus (PVN). The vagus nerve is the major outflow tract that is the calming portion of the ANS and antagonizes the PVN to lower the stress response. It has an amazing effect on the hexagonal prisms in CSF in the 4th ventricle. This signal can be turned on and off by the light that comes through the prism of our retina. This is why the light you allow is critical in signaling in how it changes hydrogen bonding in water.
Balancing both arms is critical in avoiding diseases, and creating an allostasis in optical signaling. The light in the environment destroys that balance. The autonomic nervous system tries to create order from the chaos in light. It too is another prism. The key to the orexin prism is found in its 33–amino–acid peptide sequence that has two intrachain disulfide bridges formed by four cysteine residues (C6–C12 and C7–C14). You might remember I important I told you cysteine was here.
Cysteine has something in common with orexin Cysteine is the rarest amino acid and being rare means it increases signal fidelity. Eliminating correlated noise without changing other input properties substantially decreased the accuracy with which a cell’s spike outputs encoded light inputs in retinal ganglion cells. Thus covariation of excitatory and inhibitory inputs in the orexin system can be a critical determinant of the reliability of neural coding and computation in its target in the brain.
Orexin neurons widely project to all regions of the brain including the hypothalamus (de Lecea et al., 1998; Peyron et al., 1998), thalamus, cortex, brain stem, and spinal cord (van den Pol, 1999). There aren’t many of them, but their impact on water chemistry in us defines a non-linear stimulus where a small stimulus leads to a massive change.
Orexin input to brain regions important for arousal, such as the locus ceruleus, help to regulate the response to a stressor (Hagan et al., 1999). Moreover, orexin-containing nerve terminals project to stress-sensitive centers such as the amygdala and bed nucleus of the stria terminalis (Date et al.,1999). Orexin neurons also project to the paraventricular nucleus of the hypothalamus (PVN) (Winsky-Sommerer et al., 2004), where neurons expressing corticotropin-releasing hormone (CRH = cortisol) initiate the Hypothalamic Pituitary Adrenal (HPA) Axis. This forms half of the circadian mechanism of cortisol and melatonin.
Further, orexin neurons densely project to the paraventricular nucleus of the thalamus (PVT) (Kirouac et al., 2005), which plays a role in regulating neuroendocrine and behavioral adaptations to severe or chronic stress (Hsu et al.,2014). Specifically, orexins may influence gene expression of the CRH type 1 receptor (CRH1R) in the paraventricular nucleus of the thalamus (Heydendael et al., 2012, 2011), and this brain region may then regulate the HPA axis via multisynaptic pathways through the BNST to the PVN. The orexin neuropeptides orexin A and orexin B interact with noradrenergic, cholinergic, serotonergic, histaminergic, and dopaminergic systems in the brain, in addition to the HPA axis (Sutcliffe and de Lecea, 2000). Thus, orexins have the potential to regulate the stress response through actions at multiple projection sites. All mental disorders likely begin with orexin dysfunction.
CSF-contacting neurons are present in all vertebrates and are located mainly in the hypothalamic area and the spinal cord. The hypothalamus lies below the hypothalamic sulcus separating it from the thalamus above. Like the thalamus, a thin vertical space filled with CSF called the 3rd ventricle is positioned midline between the two halves of the hypothalamus thalamus. The blood-brain barrier is absent around the vagal vomiting center of the 4th ventricle so that it can monitor the blood for changes. The blood-brain barrier is also absent around the various areas in the hypothalamus where orexin targets land their signaling.
The stimulus of light from the orexin prisms in our retina changes the CSF’s hydrogen bonding networks to a hexagonal arrangement that allows for another prism to be built in water that stores electromagnetic information to make the brain quantum coherent. Full-spectrum sunlight reaching the brain creates more coherent domains in water. Normally water is only 40% quantum coherent. From here on out you should realize that coherent domains should be regarded as long-range ensembles of electrons and protons of exclusion zones inside the water that has the superconductive ability at room temperature. This type of water is a plasma filled with electrons and protons. Biological water has unique characteristics that make life possible.
SUMMARY
Water (H2O) is the third most common molecule in the Universe (following the H2 and CO molecule), and its standard chemical structure, based on the hydrogen bond, is actually confined by a simple scheme of charges interacting via static Coulomb forces; that is, it is totally reliant on electrostatics and omits all mention of electrodynamics and the consequent radiation field. It has been speculated that a large percentage of effects in condensed matter physics make use of the radiation field in one way or another but it still doesn’t seem to have found a place in much of basic chemistry. In biochemistry, no one seems to realize how important these effects are. In biological systems almost all water is within a fraction of a micron or less from a surface or molecular backbone and so is interfacial water, which behaves in a quantum coherent manner. This occurs because as hydrogen bonds change, the Coulomb law of electrostatics does not apply to it. In these circumstances, similar charges become attractive making water react homogeneously. This water changes proteins into LED diodes capable of moving electrons around using light inside of tissues. Biology itself depends on this, so as to allow the accumulation of tissues from negatively charged cell bodies.
Biological water is paramagnetic meaning that it holds a magnetic charge.
Water has a dipole moment and is, therefore, subject to paramagnetism. Chemical bonds (including covalent, ionic, hydrogen, and van der Waals types) have been commonly assumed to be dominating for biological organization and activity. However, these bonds represent forces acting at short distances in the nanometer region. Biological systems maintain coherence at every dimension scale because of how water is changed by our molecular protein prisms in cells. Long-range coherence, large-distance cooperation, and the whole-body control are significant properties of biological systems.
According to Giuliano Preparata, Emilio Del Giudice, and colleagues water water molecules at the interface surface act like a new chemical species and can change rapidly in cells. This clustering of hydrogen bonds imparts a crystalline likeproperty to the water. It become more like a liquid crystal. In the bodies of living organisms, the clusters form hydration layers around biological molecules. Orexins are today’s example. It is known from electronics that different patterns which contain information which result within a cluster depending upon its structure of the crystal.
Thus, depending on its structure, each molecule has an oscillatory pattern (resonance frequency) that can be determined by spectroscopy. It is known, through spectrographic analysis, that water and other dipole molecules are able to be entrained to exogenous oscillatory patterns by rearranging their cluster patterns. The cluster rearrangements then resonate with the entraining frequency of sunlight to do the things life can do. This water is called a coherent domain.
Water becomes something unique when sunlight interacts with it in our cells.
Within the CD water, molecules oscillate between the ground state and an excited state close to the ionizing potential of water and, therefore, contain close to a million almost-free electrons. Those electrons are used to sculpt the physiology of life.
Quantum Electrodynamic (QED) field theory has produced a vision of water in a liquid state as a medium, which for a peculiarity of its molecular electronic spectrum reveals itself as an essential tool for long-range communications, being able to change its supra-molecular organization in the function of the interaction with the light in the environment. Orexins have the biggest prism effect of making water the stage on which life performs on.
CITES
https://www.pnas.org/doi/10.1073/pnas.0611180104
Myung J, Schmal C, Hong S, et al. The choroid plexus is an important circadian clock component. Nat Commun2018; 9: 1–13.
Xie L, Kang H, Xu Q, et al. Sleep drives metabolic clearance from the adult brain. Science 2013; 342: 373–377.
Kang J, Lim M, Bateman R, et al. Amyloid-ℬ dynamics are regulated by orexin and the sleep-wake cycle. Science2009; 326: 1005–1007.
Spector R, Snodgrass S, Johanson C. A balanced view of the cerebrospinal fluid composition and functions: focus on adult humans. Exp Neurol 2015; 273: 57–68.
Richardson SJ, Wijayagunaratne RC, D’Souza DG, et al. Transport of thyroid hormones via the choroid plexus into the brain: the role of transthyretin and thyroid hormone transmembrane transporters. Front Neurosci 2015; 9: 66.
Myung J, Wu D, Simonneaux V, et al. Strong circadian rhythms in the choroid plexus: implications for sleep-independent brain metabolite clearance. J Exp Neurosci 2018; 12: 1179069518783762.