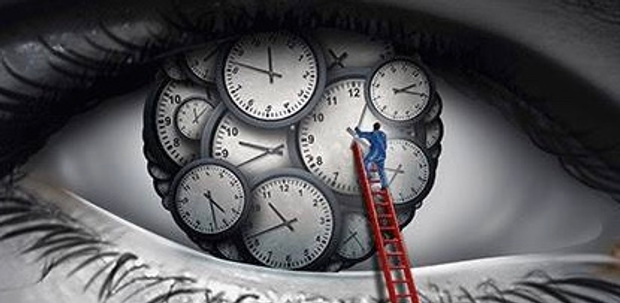
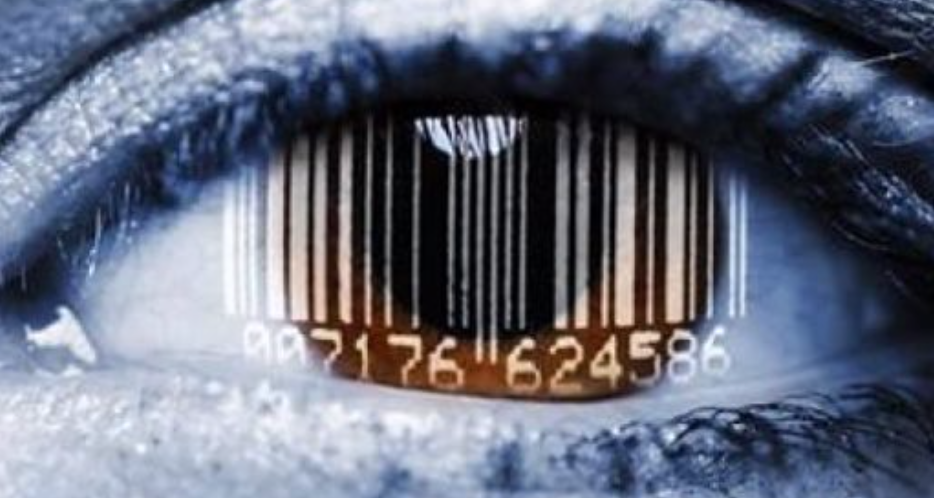
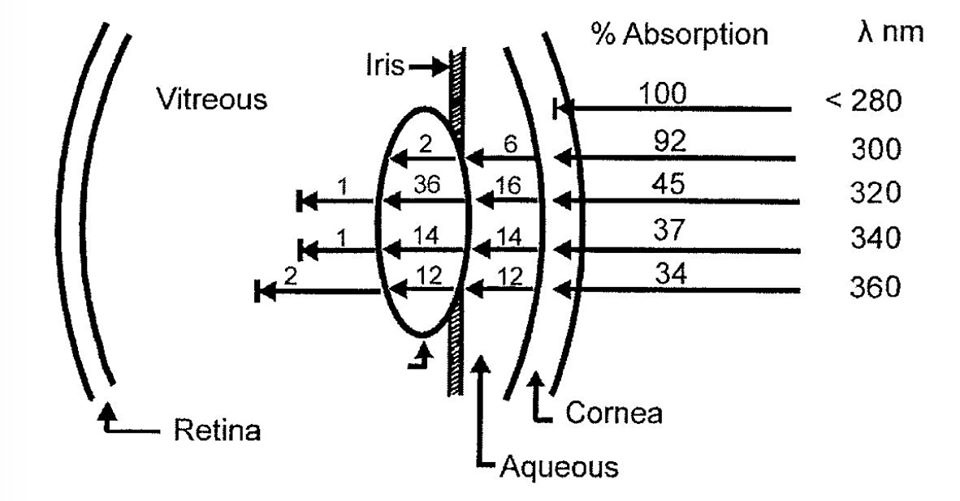
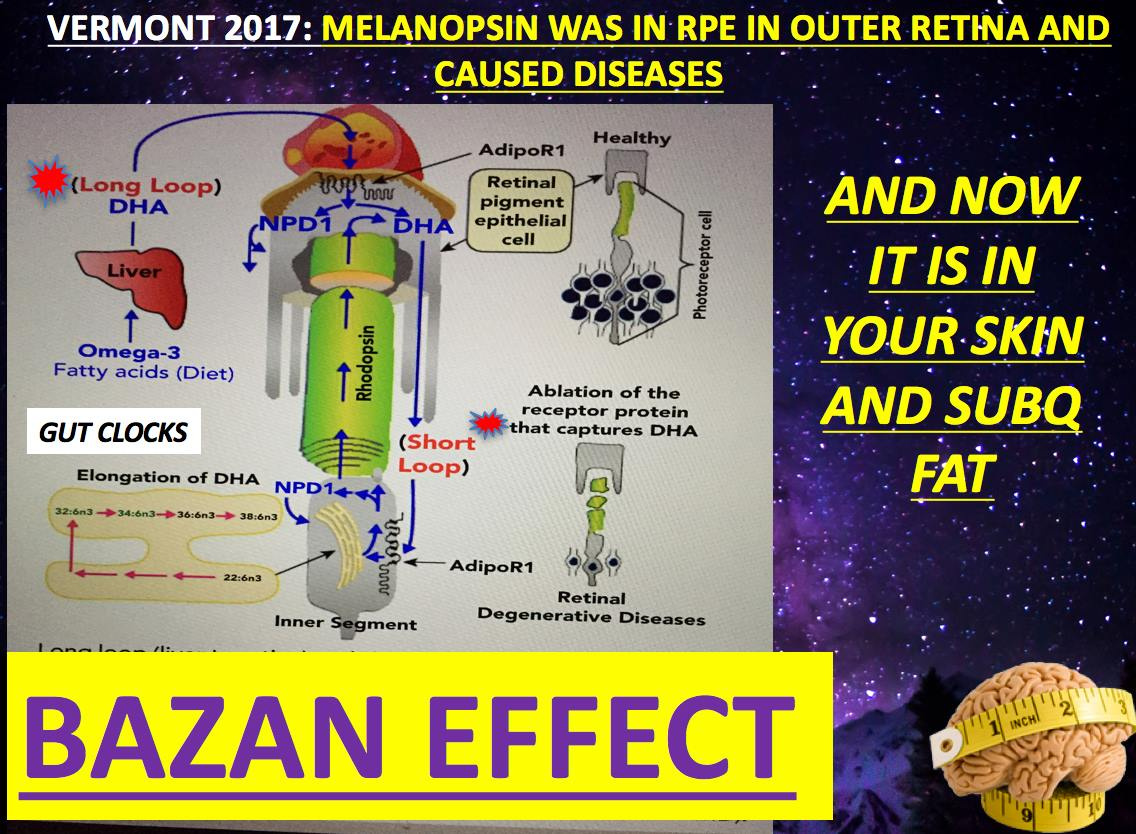
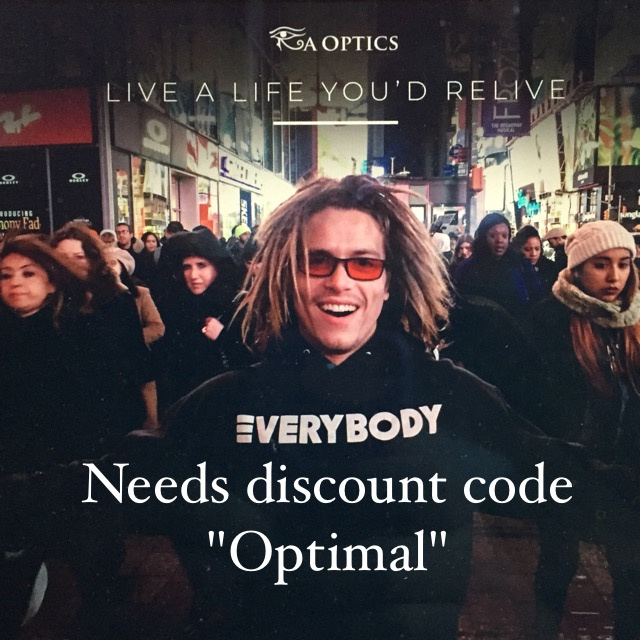
Pharmacological treatments for Alzheimer’s disease (AD) have not resulted in desirable clinical efficacy over 100 years. Hydrogen peroxide (H2O2), a reactive and the most stable compound of reactive oxygen species, contributes to oxidative stress in AD patients. What does elevated H2O2 do to neurons?
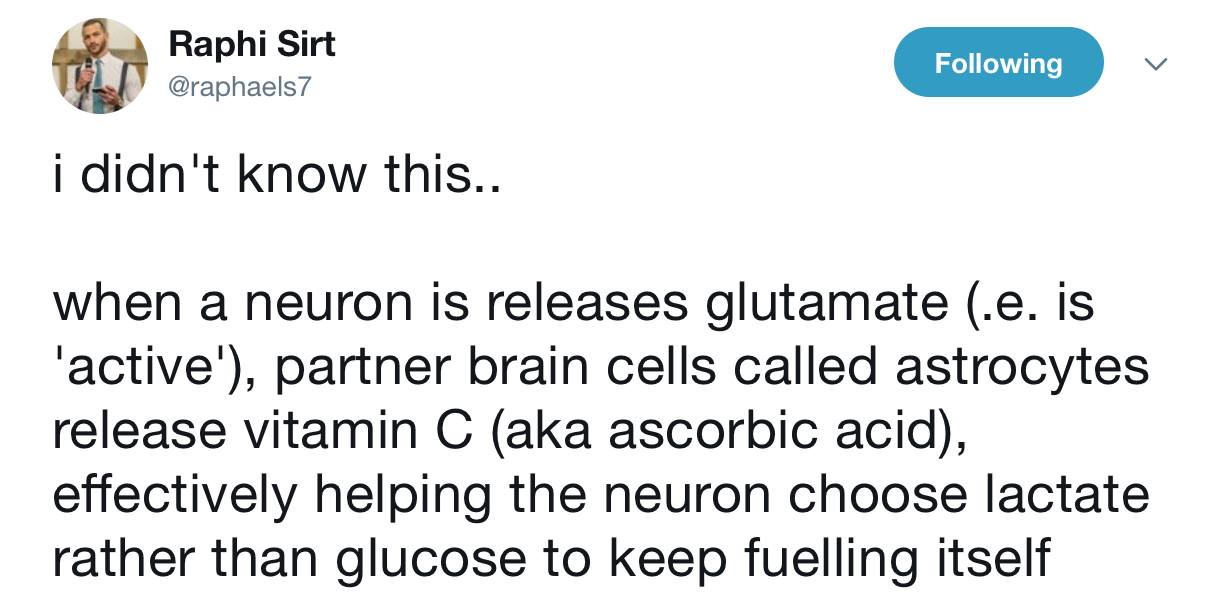
IN AD in human more glutamate is released when the environment is loaded with blue light and/or nnEMF. This in turn cascades to deplete Vitamin C.
Where did this concern come from? Brain and eye surgery where light illumination is used.
Concerns have been raised about whether operating microscopes and endo-illumination used during ophthalmic surgeries contribute to retinal damage. Despite the recognition that ascorbic acid (vitamin C) helps to protect the eye from light and the abundance of vitamin C in the retina, artificial aqueous humors used during surgery USUALLY only contain the antioxidant glutathione (GSH below).
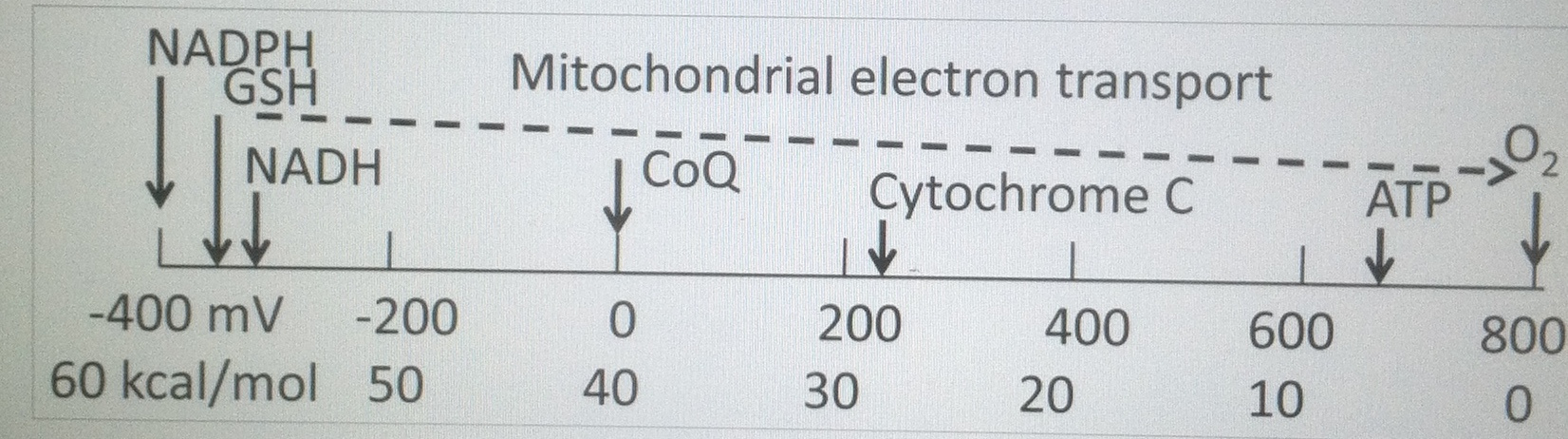
Light-induced retinal damage is irreversible and results in permanent visual loss, especially when damage involves the macular area. Ocular tissues are more sensitive and vulnerable to MAN-MADE light than other organs (Sliney, 2002). Although light-induced photoreceptor damage has been well described (Noell et al., 1966; Kuwabara and Gorn, 1968), illumination of the ocular fundus by man-made light is inevitable in clinical settings during ocular examinations and surgeries. As a large number of intraocular surgeries, such as corneal and cataract surgeries and vitrectomies, require direct microscopic illumination of ocular tissue, acute retinal damage has been increasingly observed. The damage is thought to result, at least in part, from the effects of this illumination (Arafat et al., 1994; Kuhn et al., 1991; Michels et al., 1992; Kleinmann et al., 2002). What most eye docs fail to realize is this light does not have any UV light in it, yet they continue to blame UV light for damage. If they used a spectroscope they would see that blue light is the most dominant light in artificial light and is behind the cause of eye damage during surgeries. Most experiments studying light-induced injury show retinal damage with continuous illumination over several days (Grignolo et al., 1969; Malik et al., 1986; Edward et al., 1993; Organisciak et al., 2003).
Very little UV light penetrates the surface of the globe but all of the blue light from the bulbs we use do penetrate the globe to affect the retina.
Photooxidation of light, in turn then depletes the patient’s eyes of endogenous Vitamin C and it H2O2 directly inhibits formaldehyde dehydrogenase (FDH) in neurons. FDH inactivity and semicarbazide-sensitive amine oxidase (SSAO) disorder resulted in endogenous formaldehyde (FA) accumulation. Unexpectedly, excess FA, in turn, caused acetylcholine (Ach) deficiency by inhibiting choline acetyltransferase (ChAT) activity in vitro and in vivo.
Acetylcholine (ACh) has a crucial role in the peripheral and central nervous systems. The enzyme choline acetyltransferase (ChAT) is responsible for synthesizing ACh from acetyl-CoA and choline in the cytoplasm and the vesicular acetylcholine transporter (VAChT) uptakes the neurotransmitter into synaptic vesicles. Following depolarization, ACh undergoes exocytosis reaching the synaptic cleft, where it can bind its receptors, including muscarinic and nicotinic receptors. ACh present at the synaptic cleft is promptly hydrolyzed by the enzyme acetylcholinesterase (AChE), forming acetate and choline, which is recycled into the presynaptic nerve terminal by the high-affinity choline transporter (CHT1). Cholinergic neurons located in the basal forebrain, including the neurons that form the nucleus basalis of Meynert, are severely lost in Alzheimer’s disease (AD). AD is the most ordinary cause of dementia affecting millions of people worldwide. The hallmarks of the disease are the accumulation of neurofibrillary tangles and amyloid plaques. However, there is no real correlation between levels of cortical plaques and AD-related cognitive impairment. The plaques correlate with the disease process because a falling mitochondrial redox state links to protein misfolding. Nevertheless, synaptic loss is the principal correlate of disease progression in AD and loss of cholinergic neurons contributes to memory and attention deficits.
This is why AD brains have low voltage on EEG. Levels of ACH are linked to voltages on EEG studies.
What else do the eye docs forget to account for during their surgeries?
Free radical scavengers such as glutathione (Winkler and Giblin, 1983), α-tocopherol (vitamin E) (Penn et al., 1987) and ascorbic acid (vitamin C) (Woodford et al., 1983) are thought to serve as components of an endogenous defense system that helps to limit light-induced retinal damage. Vitamin C is also abundant in the human retina, where its concentration in guinea pigs and rats is about 1.6 mM (Heath et al., 1961; Woodford et al., 1983). However, intraocular irrigating solutions currently employed during ocular surgery in humans only contain oxidized glutathione as a corneal protectant (Edelhauser et al., 1975, Araie, 1986). Importantly, these surgical solutions USUALLY do not contain vitamin C, even though human aqueous humor contains high concentrations of the vitamin.
Does this make any sense? Could this be why anterior chamber surgery has the side effect profile it does today?
The public does not know these answers because researchers rarely ask these questions in their experiments and never control for the light spectra used in surgery. How can we learn when we ask the wrong questions in our studies?
How could we measure this if we wanted too?
LDH = Lactate dehydrogenase = lactate biochemistry = the link to Vitamin C physiology of the light stress response.
LDH activity can be measured spectrophotometrically from samples of blood taken every hour during surgery. The activity of LDH can be determined by the rate at which its substrate, pyruvate, is reduced to lactate, as monitored by diminished absorbance of the reduced form of nicotinamide adenine dinucleotide (NADH) at 340 nm at cytochrome 1. If I can figure it out, why can’t they?
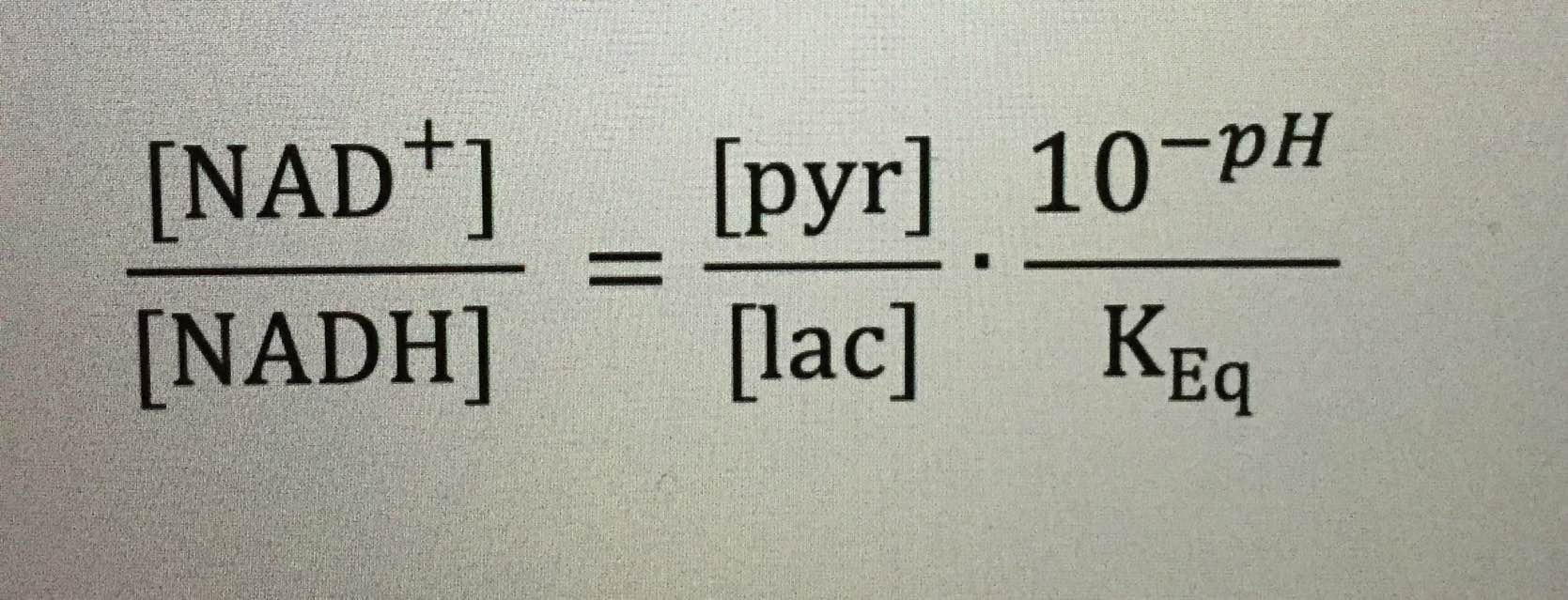
Remember the reduction of lactate implies the additions of electrons to lactate. Guess where the electrons come from in the human retina?
Vitamin C is an electron donor in human biochemistry. This implies that Vitamin C presence or absence allows a stressed cell to choose to use lactate over glucose in metabolism.
The protective effects of vitamin C are not mimicked by glutathione, an agent routinely included in surgical solutions used in eye surgery, because glutathione does not donate electrons as well in the retina (below) in biochemical reactions as Vitamin C does.
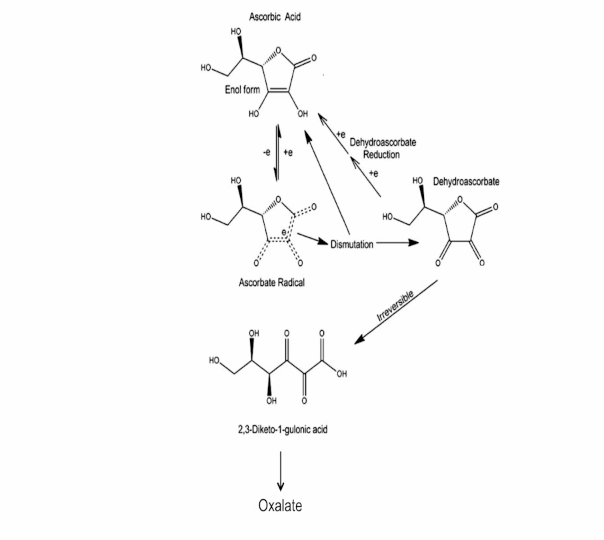
It means that ocular surgery using solutions devoid of antioxidants normally found in the retina may be driving diabetic changes in eyes and brains below our perception as surgeons.
How is that for an unintended consequence?
Following extensive light exposure in surgery, it has been found that the level of vitamin C in the retina is diminished (Woodford et al., 1983). Moreover, the fact that diurnal mammals have a normally high concentration of vitamin C in their aqueous humor but nocturnal mammals do not (Koskela et al., 1989) suggests a significant role for vitamin C in protecting diurnal animals from sunlight. Most eye research is done on nocturnal mammals so this effect magnifies the problem for humans. It should be clear now that Vitamin C does have a solar component of action (diunal actions) most never account for in their studies. Interestingly, light-induced retinal damage may be more pronounced in scorbutic than control monkeys (Tso, 1987), again suggesting the importance of vitamin C as a retinal protectant in humans and primates is reenforced.
FACTOID: What does this imply for 5G and the IoT………..needs that RFID eye chip below to control you. The bar code for the eye is terrestrial sunlight not man made parts of the electromagnetic spectrum used for telecommunications and back lit tech screens all cause these problems in your eyes.

This all points out why blue light and nnEMF are LIKELY causative in the etiology of Alzheimer’s Disease in our modern world.
2G-5G networks create the Fenton free radical in our mitochondria as the pictures in this blog show.
What does this imply for eye surgery and for AD risks longer term?
You’ve got to ask the right questions if you’re going to find the right answers. On your transition to a Black Swan, you need to ask great questions. Questions are how you uncover real truths in Nature to learn what you should and unlearn what you must.
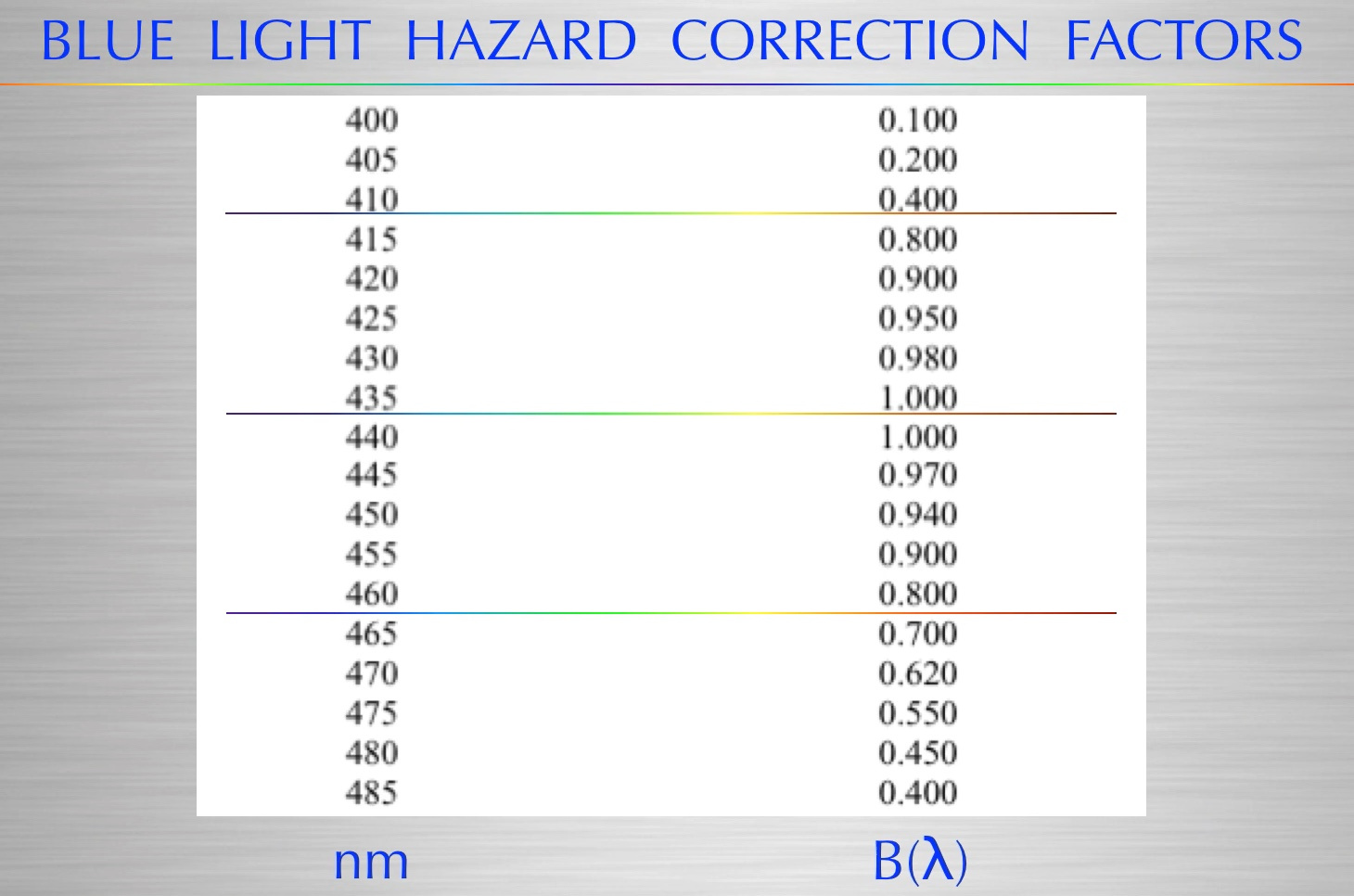
Considering the risk of phototoxicity of manmade blue lights via the blue light hazard (above)-, van den Biesen et al. (2000) warned that the safe exposure time of commercial endo-illumination is less than 11 minutes, a time frame that is unrealistic in today’s vitreoretinal surgery. Complicating this is the fact that the detached retina is even more sensitive to light (Zilis et al., 1991). Could this be why these surgeries seem to have a high failure rate and lead to more eye surgery?
These observations, coupled with the results of the present study, suggest that supplying effective free radical scavengers other than glutathione in surgical solutions may be beneficial for preventing light-induced retinal damage during intraocular surgery, especially vitrectomy, and for maintaining the physiological integrity of the retina for an extended period following surgery. The importance of carefully considering the content of surgical solutions is magnified by the fact that the following vitrectomy the retina is exposed to the ophthalmic solution for 24 hours or more, given the time it takes to replenish the 4.0 ml vitreous cavity with endogenous aqueous humor that is produced at a rate of 3.04 μl/min (Tsukamoto and Larsson, 2004).
Exogenous Vitamin C itself is not appropriate for use in surgical solutions. In the presence of ferrous ions released during bleeding, exogenous vitamin C participates in the Fenton reaction with iron liberated from bleeding (Fisher and Naughton, 2004) and this results in the production of toxic hydroxyl radicals with blue light hazard (435-465nm light).
This thread should be eye opening to any skeptic that blue light or nnEMF can cause disease in man.
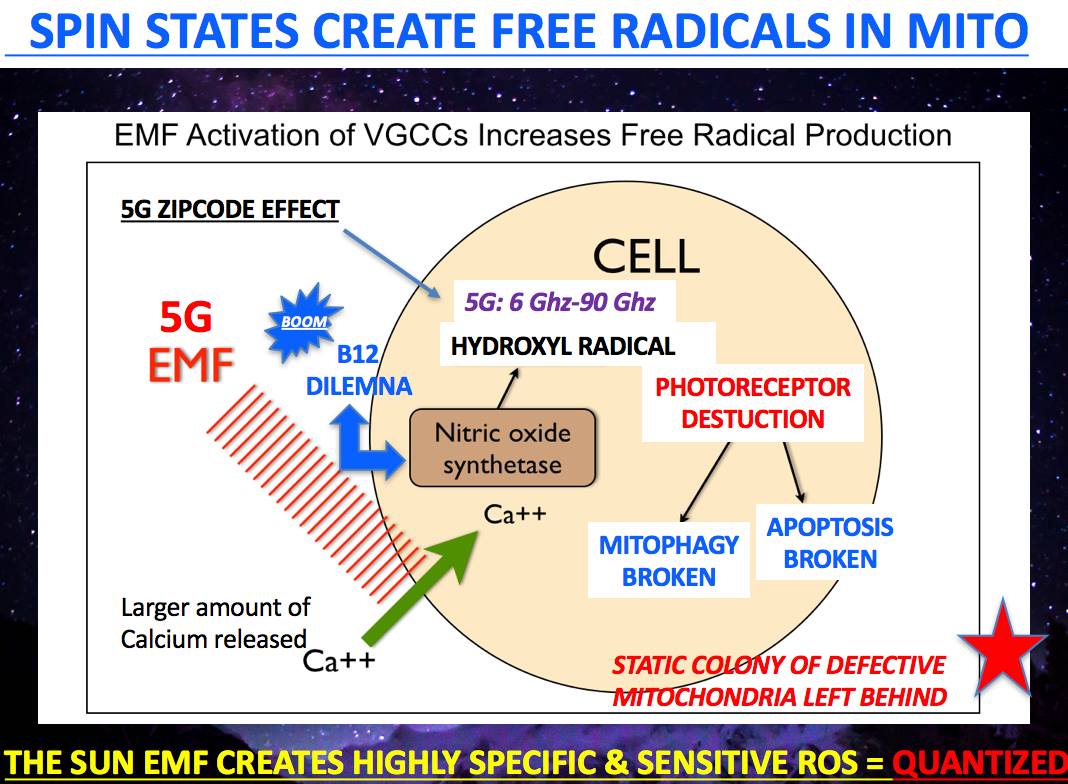
References
- An KP, Athar M, Tang X, Katiyar SK, Russo J, Beech J, Aszterbaum M, Kopelovich L, Epstein EH, Jr, Mukhtar H, Bickers DR. Cyclooxygenase-2 expression in murine and human nonmelanoma skin cancers: implications for therapeutic approaches. Photochem Photobiol. 2002;76:73–80. [PubMed] [Google Scholar]
- Arafat AF, Dutton GN, Wykes WN. Subclinical operating microscope retinopathy: the use of static perimetry in its detection. Eye. 1994;8:467–472. [PubMed] [Google Scholar]
- Araie M. Barrier function of corneal endothelium and the intraocular irrigating solutions. Arch Ophthalmol. 1986;104:435–438. [PubMed] [Google Scholar]
- Barker FM, Brainard GC, Dayhaw-Barker P. Transmittance of the human lens as a function of age. Invest Ophthalmol Vis Sci. 1991;32S:1083. [Google Scholar]
- Beatty S, Koh H, Phil M, Henson D, Boulton M. The role of oxidative stress in the pathogenesis of age-related macular degeneration. Surv Ophthalmol. 2000;45:115–134. [PubMed] [Google Scholar]
- Böhm F, Edge R, Foley S, Lange L, Truscott TG. Antioxidant inhibition of porphyrin-induced cellular phototoxicity. J Photochem Photobiol B. 2001;65:177–183. [PubMed] [Google Scholar]
- Boulton M, Rozanowska M, Rozanowski B. Retinal photodamage. J Photochem Photobiol B. 2001;64:144–161. [PubMed] [Google Scholar]
- Edelhauser HF, Van Horn DL, Hyndiuk RA, Schultz RO. Intraocular irrigating solutions. Their effect on the corneal endothelium. Arch Ophthalmol. 1975;93:648–657. [PubMed] [Google Scholar]
- Edward DP, Lim K, Sawaguchi S, Tso MO. An immunohistochemical study of opsin in photoreceptor cells following light-induced retinal degeneration in the rat. Graefes Arch Clin Exp Ophthalmol. 1993;231:289–294. [PubMed] [Google Scholar]
- Feeney L, Berman ER. Oxygen toxicity: membrane damage by free radicals. Invest Ophthalmol. 1976;15:789–792. [PubMed] [Google Scholar]
- Fisher AE, Naughton DP. Iron supplements: the quick fix with long-term consequences. Nutr J. 2004;16:2. [PMC free article] [PubMed] [Google Scholar]
- Grignolo A, Orzalesi N, Castellazzo R, Vittone P. Retinal damage by visible light in albino rats. An electron microscope study. Ophthalmologica. 1969;157:43–59. [PubMed] [Google Scholar]
- Grimm C, Wenzel A, Williams T, Rol P, Hafezi F, Reme C. Rhodopsin-mediated blue-light damage to the rat retina: effect of photoreversal of bleaching. Invest Ophthalmol Vis Sci. 2001;42:497–505.[PubMed] [Google Scholar]
- Ham WT, Jr, Mueller HA, Ruffolo JJ, Jr, Guerry D, 3rd, Guerry RK. Action spectrum for retinal injury from near-ultraviolet radiation in the aphakic monkey. Am J Ophthalmol. 1982;93:299–306.[PubMed] [Google Scholar]
- Heath H, Beck TC, Rutter AC. Biochemical changes in aphakia. Vision Res. 1961;1:274–286.[Google Scholar]
- Izumi Y, Benz AM, Kirby CO, Labruyere J, Zorumski CF, Price MT, Olney JW. An ex vivo rat retinal preparation for excitotoxicity studies. J Neurosci Methods. 1995;60:219–225. [PubMed] [Google Scholar]
- Izumi Y, Hammerman SB, Kirby CO, Benz AM, Olney JW, Zorumski CF. Involvement of glutamate in ischemic neurodegeneration in isolated retina. Vis Neurosci. 2003;20:97–107. [PubMed] [Google Scholar]
- Keino H, Mimura S, Nagae H, Banno T, Kashiwamata S. Protection by L-ascorbic acid against phototoxicity in tin-protoporphyrin-treated suckling rats. Biol Neonate. 1993;63:183–190. [PubMed] [Google Scholar]
- Kleinmann G, Hoffman P, Schechtman E, Pollack A. Microscope-induced retinal phototoxicity in cataract surgery of short duration. Ophthalmology. 2002;109:334–338. [PubMed] [Google Scholar]
- Koskela TK, Reiss GR, Brubaker RF, Ellefson RD. Is the high concentration of ascorbic acid in the eye an adaptation to intense solar irradiation? Invest Ophthalmol Vis Sci. 1989;30:2265–2267.[PubMed] [Google Scholar]
- Kuhn F, Morris R, Massey M. Photic retinal injury from endoillumination during vitrectomy. Am J Ophthalmol. 1991;111:42–46. [PubMed] [Google Scholar]
- Kuwabara T, Gorn RA. Retinal damage by visible light. An electron microscopic study. Arch Ophthalmol. 1968;79:69–78. [PubMed] [Google Scholar]
- Li J, Edward DP, Lam TT, Tso MO. Amelioration of retinal photic injury by a combination of flunarizine and dimethylthiourea. Exp Eye Res. 1993;56:71–78. [PubMed] [Google Scholar]
- Li ZY, Tso MO, Wang HM, Organisciak DT. Amelioration of photic injury in rat retina by ascorbic acid: a histopathologic study. Invest Ophthalmol Vis Sci. 1985;26:1589–1598. [PubMed] [Google Scholar]
- Lisby S, Gniadecki R, Wulf HC. UV-induced DNA damage in human keratinocytes: quantitation and correlation with long-term survival. Exp Dermatol. 2005;14:349–355. [PubMed] [Google Scholar]
- Malik S, Cohen D, Meyer E, Perlman I. Light damage in the developing retina of the albino rat: an electroretinographic study. Invest Ophthalmol Vis Sci. 1986;27:164–167. [PubMed] [Google Scholar]
- Marchioli R. Antioxidant vitamins and prevention of cardiovascular disease: laboratory, epidemiological and clinical trial data. Pharmacol Res. 1999;40:227–238. [PubMed] [Google Scholar]
- Merriam JC, Löfgren S, Michael R, Söderberg P, Dillon J, Zheng L, Ayala M. An action spectrum for UV-B radiation and the rat lens. Invest Ophthalmol Vis Sci. 2000;41:2642–2647. [PubMed] [Google Scholar]
- Michels M, Lewis H, Abrams GW, Han DP, Mieler WF, Neitz J. Macular phototoxicity caused by fiberoptic endoillumination during pars plana vitrectomy. Am J Ophthalmol. 1992;114:287–296.[PubMed] [Google Scholar]
- Noell WK, Walker VS, Kang BS, Berman S. Retinal damage by light in rats. Invest Ophthalmol. 1966;5:450–473. [PubMed] [Google Scholar]
- Noell WK. Possible mechanisms of photoreceptor damage by light in mammalian eyes. Vision Res. 1980;20:1163–1171. [PubMed] [Google Scholar]
- Organisciak DT, Darrow RM, Barsalou L, Kutty RK, Wiggert B. Susceptibility to retinal light damage in transgenic rats with rhodopsin mutations. Invest Ophthalmol Vis Sci. 2003;44:486–492.[PubMed] [Google Scholar]
- Park CH, Amare M, Savin MA, Hoogstraten B. Growth suppression of human leukemic cells in vitro by L-ascorbic acid. Cancer Res. 1980;40:1062–1065. [PubMed] [Google Scholar]
- Penn JS, Naash MI, Anderson RE. Effect of light history on retinal antioxidants and light damage susceptibility in the rat. Exp Eye Res. 1987;44:779–788. [PubMed] [Google Scholar]
- Pentland AP, Schoggins JW, Scott GA, Khan KN, Han R. Reduction of UV-induced skin tumors in hairless mice by selective COX-2 inhibition. Carcinogenesis. 1999;20:1939–1944. [PubMed] [Google Scholar]
- Postel EA, Pulido JS, Byrnes GA, Heier J, Waterhouse W, Han DP, Mieler WF, Guse C, Wipplinger W. Long-term follow-up of iatrogenic phototoxicity. Arch Ophthalmol. 1998;116:753–757.[PubMed] [Google Scholar]
- Prasad KN, Sinha PK, Ramanujam M, Sakamoto A. Sodium ascorbate potentiates the growth inhibitory effect of certain agents on neuroblastoma cells in culture. Proc Natl Acad Sci U S A. 1979;76:829–832. [PMC free article] [PubMed] [Google Scholar]
- Romano C, Price MT, Olney JW. Delayed excitotoxic neurodegeneration induced by excitatory amino acid agonists in isolated retina. J Neurochem. 1995;65:59–67. [PubMed] [Google Scholar]
- Schmidt RE, Zuclich JA. Retinal lesions due to ultraviolet laser exposure. Invest Ophthalmol Vis Sci. 1980;19:1166–1175. [PubMed] [Google Scholar]
- Sliney DH. How light reaches the eye and its components. Int J Toxicol. 2002;21:501–509.[PubMed] [Google Scholar]
- Taylor A, Jacques PF, Nadler D, Morrow F, Sulsky SI, Shepard D. Relationship in humans between ascorbic acid consumption and levels of total and reduced ascorbic acid in lens, aqueous humor, and plasma. Curr Eye Res. 1991;10:751–759. [PubMed] [Google Scholar]
- Tokuda K, Tsukamoto T, Fujisawa S, Matsubara M. Evaluation of toxicity due to vital stains in isolated rat retinas. Acta Ophthalmol Scand. 2004;82:189–194. [PubMed] [Google Scholar]
- Tso MO. Retinal photic injury in normal and scorbutic monkeys. Trans Am Ophthalmol Soc. 1987;85:498–556. [PMC free article] [PubMed] [Google Scholar]
- Tsukamoto H, Larsson LI. Aqueous humor flow in normal human eyes treated with brimonidine and dorzolamide, alone and in combination. Arch Ophthalmol. 2004;122:190–193. [PubMed] [Google Scholar]
- van den Biesen PR, Berenschot T, Verdaasdonk RM, van Weelden H, van Norren D. Endoillumination during vitrectomy and phototoxicity thresholds. Br J Ophthalmol. 2000;84:1372–1375. [PMC free article] [PubMed] [Google Scholar]
- Winkler BS, Giblin FJ. Glutathione oxidation in retina: effects on biochemical and electrical activities. Exp Eye Res. 1983;36:287–297. [PubMed] [Google Scholar]
- Woodford BJ, Tso MO, Lam KW. Reduced and oxidized ascorbates in guinea pig retina under normal and light-exposed conditions. Invest Ophthalmol Vis Sci. 1983;24:862–867. [PubMed] [Google Scholar]
- Wu J, Seregard S, Spangberg B, Oskarsson M, Chen E. Blue light induced apoptosis in rat retina. Eye. 1999;13:577–583. [PubMed] [Google Scholar]
- Zhang C, Lei B, Lam TT, Yang F, Sinha D, Tso MO. Neuroprotection of photoreceptors by minocycline in light-induced retinal degeneration. Invest Ophthalmol Vis Sci. 2004;45:2753–2759.[PubMed] [Google Scholar]
- Zilis JD, Machemer R. Light damage in detached retina. Am J Ophthalmol. 1991;111:47–50.[PubMed] [Google Scholar]
- http://www.ccmu.edu.cn/docs/20180912110556617083.pdf
- https://www.ncbi.nlm.nih.gov/pmc/articles/PMC1939862/